- News
- Overview
- Research
- Related Links
News
|
Mathematicians and geochemists have long realized that compositional data intrinsically exhibit a structure prone to spurious and induced correlations. This paper demonstrates, using the Na–Cl–Br system, that these mathematical problems are exacerbated in the study of sedimentary basin brines by... |
Thursday, December 13, 2012 Type: Outside Publication
|
|
This study presents geostatistical simulations of coal-quality parameters, major oxides and trace metals for an area covering roughly 812 km2 of the Blue Gem coal bed in southeastern Kentucky, USA. The Blue Gem, characterized by low ash yield and low sulfur content, is an important economic resource... |
Thursday, November 08, 2012 Type: Outside Publication
|
|
The Organic Petrology Laboratory (OPL) of the U.S. Geological Survey (USGS) Eastern Energy Resources Science Center in Reston, Virginia, contains several thousand processed coal sample materials that were loosely organized in laboratory drawers for the past several decades... |
Thursday, September 27, 2012 Type: Publication
|
|
Samples of natural gas were collected as part of a study of formation water chemistry in oil and gas reservoirs in the Appalachian Basin. Nineteen samples (plus two duplicates) were collected from 11 wells producing gas from Upper Devonian sandstones and the Middle Devonian Marcellus Shale... |
Monday, July 30, 2012 Type: Publication
|
|
Groundwater concentration data and solid-phase leachate data were collected for samples taken in and around Tuba City Open Dump, Tuba City, Arizona, in 2008. Sample locations, sample collection and analytical methods, and previous geochemical results are provided in Johnson and others (2008)... |
Monday, June 25, 2012 Type: Publication
|
|
Electron beam microanalysis of coal samples in U.S. Geological Survey (USGS) labs confirms that As is the most abundant minor constituent in Fe disulfides in coal and that Se, Ni, and other minor constituents are present less commonly and at lower concentrations than those for As. |
Tuesday, May 01, 2012 Type: Outside Publication
|
|
USGS Scientific Investigations Report: Gas Hydrate Prospecting Using Well Cuttings and Mud-Gas Geochemistry from 35 Wells, North Slope, Alaska. Part of a USGS and Bureau of Land Management gas hydrate research collaboration. |
Sunday, February 12, 2012 Type: Publication
|
|
Despite the growing interest in using energy sources other than liquid hydrocarbons (e.g., electricity, natural gas, hydrogen) in the transportation sector, it will be decades before widespread, substantive change to our energy and transportation infrastructure can be implemented... |
Monday, December 26, 2011 Type: Outside Publication
|
|
The principal mission of the USGS Energy Resources Program is to (1) understand the processes critical to the formation, accumulation, occurrence, and alteration of geologically based energy resources; (2) conduct scientifically robust assessments of those resources; and (3) study the impacts of... |
Sunday, December 04, 2011 Type: Publication
|
|
There is increased concern about the future availability of rare earth elements (REE) because of China’s dominance as the supplier of more than 95 percent of world REE output, their decision to restrict exports of rare earth... |
Wednesday, September 14, 2011 Type: Publication
|
Overview
Geochemistry research has been conducted under the Petroleum Processes Research Project since 1995. The project objectives are to apply and provide cutting-edge research on processes related to petroleum (oil, gas, and asphalt) generation, migration, and entrapment, and the consequences of these processes on petroleum type, quantity, quality, and location. The research results strengthen the scientific credibility of realizing and assessing undiscovered conventional and unconventional petroleum resources at a national and global level and are critical to formulating a better understanding and strategic use of petroleum resources.
Major research topics include origins of petroleum, genetic petroleum correlations, natural gas studies, shale gas, sedimentary basin modeling, and microbial natural gas.

Figure 1. An illustration of a hydrous pyrolysis experimental method used to simulate natural petroleum generation in the laboratory.

Photo: A close-up photographic view of a hydrous pyrolysis experimental method used to
simulate natural petroleum generation in the laboratory.
Top of Page
Research
Basin Modeling
The petroleum industry now ranks basin modeling as the top leadership technology that increases exploration success and lowers finding costs. Over the last decade, three-dimensional (3D) imaging and modeling of the subsurface through time have co-evolved and emerged as a major research focus of the petroleum industry. Virtually all major oil companies have independently recognized the need for... [+]
- Organize data, allowing deficiencies or inconsistencies to be identified
- Archive data (data loss due to personnel attrition and reorganization is a major cost factor)
- Facilitate visualization of geologic processes and communication with stakeholders
- Add value by converting static data into dynamic processed data and interpretations and
- Require many disciplines to work toward a single goal.
Major application of 3D petroleum systems models can serve as evolving databases that provide surface and subsurface geologic information for various practical research needs. They allow users to figuratively look within the earth to examine data, appraise the reliability of geological concepts, models, or geochemical input, and extract needed information. Major applications of 3D geologic models include developing predictive exploration and reservoir models, integrating sequence stratigraphy and assessment units, predicting the extent and timing of petroleum generation in source rocks, structural deformation that disturbs basin architecture, migration pathways, and locations of potential traps and accumulations, and analysis of risk based on different geologic, geochemical, or fluid-flow assumptions. Thus, 3D petroleum system models can provide a basic geoscience framework to conduct and record a wide variety of applied and basic research.
As petroleum becomes more difficult to find and reserves become more difficult to replace, 3D petroleum systems modeling has grown because it better quantifies the generation, migration, and entrapment of the remaining resource. It also facilitates interpretation of the stratigraphic and sedimentologic processes that are important to develop a predictive sequence stratigraphic framework. Because of financial and time constraints, large oil companies typically conduct 3D modeling studies only at scales to suit their immediate needs. These studies commonly cover only the acreage held by each company and seldom cover the full extent of each petroleum system. This knowledge gap represents an opportunity for the USGS because many domestic and international companies are willing to supply data and expertise. Companies will benefit from USGS studies that extend 3D interpretations beyond immediate concession areas. For example, many oil companies exhaustively study “postage-stamp” areas within basins, but 3D modeling may not have been conducted over the entire area (e.g., Gulf of Mexico, San Joaquin, Permian, Los Angeles basins, North Caspian Basin).
3D petroleum systems modeling is rapidly growing as a tool to better understand subsurface migration, accumulation, and preservation. The approach is strong as a tool to predict the pod of active source rock, thermal maturity of the source rock, migration pathways, and the timing of petroleum generation. 3D modeling is currently less successful as a means to predict volumes of trapped petroleum, their detailed compositions, or the effects of secondary processes. However, solutions to these questions could have major impact on the domestic and world economy. 3D modeling is a tool that will continue to attract new users because of the potential for high-impact solutions to these problems with respect to exploration, development, and assessment.

Figure 1. Input geometry for a 3D North Slope, Alaska model. The study area polygon shows the position and surface shape of the 3D model (top). The 2D cross section along the key E-W wells (white vertical lines; bottom) was cut from the 3D input model. The 2D cross section was used to reconstruct the shape, current thickness, and eroded thickness of the prograding foresets in the Brookian section. The pre-erosional thickness (maximum burial depth) was determined using a linear extrapolation of measured vitrinite reflectance (Ro) on semilog scale for each well. Reconstructed lost overburden reaches up to ~4000 m in the western part of the section. This lost section controls the burial depth and thermal maturity of the source rock and geometry of the migration paths.

Figure 2. Simplified perspective view of a 3D North Slope model (note north arrow) is based on layered surfaces derived from well and seismic data. Rather than using a stratigraphic subdivision of the Brookian clinoform sequences, timelines of eastward prograding foresets were mapped to allow for a time-transgressive change of geometry. LCU = Lower Cretaceous unconformity.
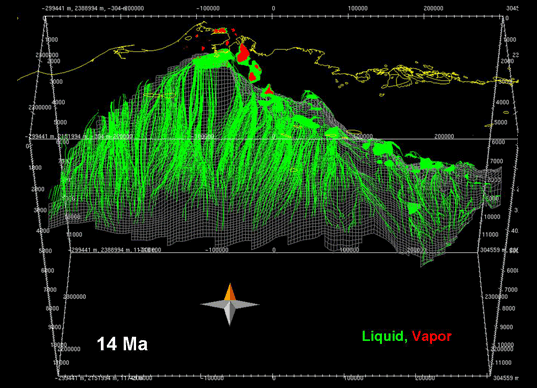
Figure 3. Three-dimensional numerical models allow predictions of petroleum migration pathways and accumulations through time. Example shows predicted migration pathways and accumulations (green and red for liquid and vapor, respectively) on the North Slope above the Triassic Shublik Formation source rock (hatched gray) 14 million years ago based on a calibrated petroleum systems model created using PetroMod® software.
Genetic Correlations
Determining genetic petroleum families and their correlation with source rocks are critical to defining petroleum systems and interpreting the processes that are controlling the generation, migration and accumulation of petroleum within sedimentary basins. Geochemical parameters based predominantly on biomarker technology have been established to genetically correlate crude oils and their sources... [+]
and aid in defining petroleum systems (see Peters et al., 2005). However, crude oil is not the only petroleum product exploited in sedimentary basin; condensates and gases are also significant. In order to more thoroughly and completely understand the dynamic petroleum processes operating within sedimentary basins, reliable and scientifically sound geochemical parameters are needed to perform (1) condensate-source rock and condensate-oil correlations, (2) gas-source correlations, and (3) identify and quantify mixing of genetically unrelated crude oils, natural gas and condensates. The absence of biomarkers in most condensates precludes the interpretation of the origin of condensates, correlation with sources and the identification of mixtures using established methods. Thus, new tools that integrate molecular and isotopic data to interpret source facies and mixing processes are required. Previous studies have attempted to decipher mixed petroleum systems (Peters and others, 1989; Jiang and Li, 2002; Chen and others, 2003) with some success. However, challenges remain due to the effects of maturity, source rock facies variation, secondary alteration, and migration fractionation. Currently, no established methodology exists to unequivocally differentiate these mixtures. The issue of differentiating crude oils that represent genetic end-members and varying mixtures is particularly important in determining total petroleum systems and their assessment units.
Gas-rich petroleum systems are receiving more attention, as natural gas becomes a more dominant component of our energy mix. Currently, characterizing natural gases is limited to molecular concentrations and stable isotopes of C1 to C5 hydrocarbons. Condensates commonly coexist with natural gases in many accumulations, but correlation of this type of petroleum with natural gases and crude oils to define petroleum systems and assessment units that are dominated by natural gas and condensate is in the early stages of development (Gurgey et al., 2005). Figure 1 (Gurgey et al. 2005) demonstrates how isotopic data can be used to correlate gases and condensates with oils. Integration of genetic molecular interpretations with this type of isotopic data will enhance petroleum system and process interpretation capabilities.

Figure 1: Correlation of condensates and gases with oils based on isotopic data (From Gurgey et al., 2005).
Migration distance/pathways, thermal maturity, and reservoir alteration can affect the geochemical parameters used to correlate natural gas, condensate, and crude oil to their source rock. A robust suite of geochemical parameter parameters that are not affected by these geological factors remains to be established to unequivocally determine the sources of genetically related natural gases, condensates, and crude oils of a petroleum system.
Determining genetic petroleum families and their correlation with source rocks are critical to defining petroleum systems and interpreting the processes that are controlling the generation, migration and accumulation of petroleum within sedimentary basins. Although a variety of geochemical parameters are currently available for correlating oils to oils and their source rocks, there is a definite need to establish geochemical parameters to correlate gas and condensate to one another and to their related oils and source rocks. Results from this research are essential in defining petroleum systems that are gas or oil dominated.
Microbial Natural Gas
Natural gas generated from microbial activity in natural organic deposits (coal, black shale, petroleum) represents an increasingly important natural resource. In the past, producers have tended to ignore microbial-derived natural gas deposits because they were considered too small to be economic. However, the increasing demand for natural gas has encouraged producers to begin targeting these smaller... [+]
smaller microbial natural gas deposits. It is estimated that natural gas from microbial activity (methanogenesis) accounts for about 20% of the world's natural gas resource. Since this gas is biologically produced, it also represents a possible renewable resource. Examples of microbial-produced natural gas deposits include: the organic-rich Antrim shale deposits in northern Michigan, and the shallow eastern edge of the Powder River Basin coal in Wyoming. Significant coal-bed gas resources may also exist in subsurface Wilcox Group and younger (Paleocene-Eocene) coal beds found across much of the Gulf Coastal Plain, and preliminary geochemical and isotopic work by USGS and others has shown that Wilcox coal gas in north Louisiana originates from microbial methanogenesis.
Although a considerable body of research exists on the biology of methanogenesis, there is much less known about the microbial-mediated conversion of geopolymers such as coal, black shale, and petroleum to methane. Methanogenesis involves a large consortium of microorganisms in order to convert the geopolymers in fossil fuels to methane (Figure 1). Methanogenic archaea are the end producers of methane, but the consortia also includes fermenting bacteria that biodegrade geopolymers in the organic deposit to simpler molecules utilized by methanogenic archaea. The nature of the microorganisms, enzyme systems, and decomposition pathways involved in the production of microbial natural gas from organic deposits is actually poorly understood. This task will examine the process involved in microbial production of methane from organic deposits using both field studies and laboratory experiments.
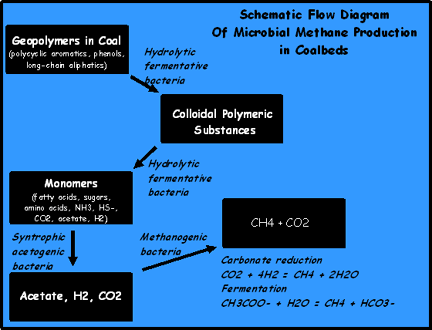
Figure 1. Conceptual model of microbial conversion of geopolymeric carbon to methane.
Organic Origins of Petroleum
Organic Origins of Petroleum
Petroleum is a naturally occurring substance consisting of organic compounds in the form of gas, liquid, or semisolid. Organic compounds are carbon molecules that are bound to hydrogen (e.g., hydrocarbons) and to a lesser extent sulfur, oxygen, or nitrogen. The simplest of these compounds is methane with one carbon atom bound to four hydrogen atoms (Figure 1). Asphaltenes are the most complex with more than... [+]
136 carbon atoms bound to more than 167 hydrogen atoms, 3 nitrogen atoms, 2 oxygen atoms, and 2 sulfur atoms (Figure 1). Petroleum gas is referred to as natural gas, which should not be confused with the abbreviated term used to describe the refined fuel "gasoline". Natural gas consists predominantly of simple hydrocarbons with only one to five carbon atoms (i.e., methane to pentane, respectively, Figure 1). Liquid petroleum is referred to as crude oil and consists of a wide range of more complex hydrocarbons and minor quantities of asphaltenes (Figure 1). Semisolid petroleum is tar, which is dominated by larger complex hydrocarbons and asphaltenes (Figure 1).
Figure 1. Some examples of organic compounds in petroleum, from the simplest (methane) to the most complex (asphaltene).
Petroleum formation takes place in sedimentary basins, which are areas where the Earth's crust subsides and sediments accumulate within the resulting depression. As the sedimentary basin continues to subside, sediment accumulations continue to fill the depression. This results in a thickening sequence of sediment layers in which the lower sediment layers eventually solidify into sedimentary rocks as they experience greater pressures and temperatures with burial depth. The sediment layers that accumulate vary in character because the sources and depositional settings of the sediments change through geologic time as the sedimentary basin subsides and fills. It is critical to petroleum formation that at some time during the accumulation of sediments at least one of the sediment layers contains the remains of deceased plants or microorganisms. Throughout geologic time, the world oceans have expanded and receded over the Earth’s land surfaces and contributed sediment layers to subsiding sedimentary basins. Development of stagnant water conditions in some of the expanded oceans caused the bottom waters to be depleted in oxygen (anoxic), which allowed portions of decaying plankton (e.g., algae, copepods, bacteria, and archaea) that originally lived in the upper oxygen-bearing (oxic) waters to be preserved as a sediment layer enriched in organic matter (Figure 2). Swamps and marshes may also develop marginal to oceans overlying subsiding basins. In these depositional settings, sediment layers enriched in decaying land plants (e.g., trees, shrubs, and grasses) may occur.
As these organic-rich sediment layers are buried by deposition of overlying sediments in the subsiding basin, the sediments are compressed and eventually lithified into rocks referred to as black shale, bituminous limestone, or coal. Methane producing microorganisms referred to as methanogens may thrive under certain favorable conditions within the organic-rich sediment layer during its early burial. These microorganisms consume portions of the organic matter as a food source and generate methane as a byproduct. This methane, which is typically the main hydrocarbon in natural gas, has a distinct neutron deficiency in its carbon nuclei (i.e., carbon isotopes), which allows microbial natural gas (a.k.a., biogenic gas) to be readily distinguished from methane generated by thermal processes (a.k.a., thermogenic gas) later in a basin's subsidence history. The microbial methane may remain in the organic-rich layer or it may bubble up into the overlying sediment layers and escape into the ocean waters or atmosphere. If impermeable sediment layers, called seals, hinder the upward migration of microbial gas, the gas may collect in underlying porous sediments, called reservoirs (Figure 3).
Figure 2: Formation of organic-rich sediment
layer.
Figure 3: Early burial of sediment layers in
basin.
Economically significant accumulations of microbial natural gas have been estimated to account for 20 percent of the world’s produced natural gas. Microbial methane may remain trapped in the organic-rich sediment layer through out its lithificaton and contribute to economic accumulations referred to as coal-bed methane and shale gas.
Burial of the organic-rich rock layer may continue in some subsiding basins to depths of 6,000 to 18,000 feet (1830 to 5490 m).At these depths, the organic-rich rock layer is exposed to temperatures of 150 to 350 ºF (66 to 177 ºC) for a few million to tens of millions of years. The organic matter within the organic-rich rock layer begins to cook during this period of heating and portions of it thermally decompose into crude oil and natural gas (i.e., thermogenic gas) (Figure 4).
This overall process of cooking petroleum out of an organic-rich rock layer involves the appropriate combination of temperature and time and is referred to as thermal maturation. If the original source of the organic matter is mostly higher plants (e.g., trees, shrubs, and grasses), natural gas will be the dominant petroleum generated with lesser amounts of crude oil generation. If the original source of the organic matter is plankton (e.g., algae, copepods, and bacteria), crude oil will be the dominant petroleum generated with lesser amounts of natural gas generation. Organic-rich rock layers that have undergone this process of petroleum generation are considered to be thermally mature and referred to as source rocks.
Organic-rich rocks that have not been thermally matured are referred to as being thermally immature. These immature organic-rich rocks may be referred to as oil shale if artificial heating at high temperatures (~1000ºF/~538ºC) in surface or near-surface reactors (a.k.a., retorts) yield economic quantities of oil. Oil shale retorting occurred in Scotland between 1860 and 1960 and is currently active in Estonia and Brazil.
Petroleum has a lower density than the water that occupies pores, voids, and cracks in the source rock and the overlying rock and sediment layers. This density difference forces the generated petroleum to migrate upwards by buoyancy until sealed reservoirs in the proper configurations serve as traps that concentrate and collect the petroleum. Some of the generated natural gas may not migrate out and away from its source rock, but instead remains within microscopic pores and dissolved in the organic matter of its source rock. This retained natural gas has proven to be an economically significant resource that is referred to as shale gas. The Barnett Shale in the Fort Worth basin of Texas is a good example of this type of accumulation.
In some basins, petroleum may not encounter a trap and continue migrating upward into the overlying water or atmosphere as petroleum seeps. Crude oil that migrates to or near the surface of a basin will lose a considerable amount of its hydrocarbons to evaporation, water washing, and microbial degradation leaving a residual tar enriched in large complex hydrocarbons and asphaltenes (Figure 5). Tar deposits range in size from small local seeps like the La Brea tar pits of California to regionally extensive occurrences as observed in the Athabasca tar sands of Alberta.
Figure 4: Continued burial of sediment and rock
layers in subsiding basin.
Figure 5: Deeper burial of rock layers in
subsiding basin.
Burial of the source rock may continue to depths greater than 20,000 ft. (6100 m) in some sedimentary basins. At these depths, temperatures in greater than 350ºF (177ºC) and pressures greater than 15,000 psi (103 MPa) transform the remaining organic matter into more natural gas and a residual carbon referred to as char. Oil trapped in reservoirs that are sometimes buried to these depths also decomposes to natural gas and char. The char, which is also called pyrobitumen, remains in the original reservoir while the generated natural gas may migrate upward to shallower traps within the overlying rock layers of the basin. The Gulf Coast basin that extends into the offshore of Louisiana and the Anadarko basin of the US mid-continent are good examples of these deep basins.
Further burial to temperatures and pressures in excess of 600ºF (316ºC) and 60,000 psi (414 MPa), respectively, represent metamorphic conditions in which the residual char converts to graphite with the emission of molecular hydrogen gas. The resulting metamorphic rocks are graphitic slate, schist or marble. Thermodynamic considerations indicate that water remaining in these rocks should react with the graphite to form either methane or carbon dioxide depending on the amount of molecular hydrogen present. Currently, the deepest wells in sedimentary basins do not exceed 32,000 ft (9760 m). Therefore, the significance of natural gas generation under these extreme conditions remains uncertain.
Sedimentary basins vary considerably in size, shape, and depth all over the Earth’s crust (Figure 6).
Figure 6: General outline of major sedimentary basins.
A large number of variables and different combinations of these variables determine whether a sedimentary basin contains microbial methane, natural gas, crude oil, tars, or no petroleum. Not all basins have organic-rich sediment layers deposited during their subsidence history. As a result, these basins will contain no appreciable quantities of petroleum regardless of how deep the basin subsides. Other basins that do have an organic-rich rock layer may not have been buried to sufficient depths to generate natural gas or crude oil through thermal maturation, but may contain microbial methane accumulations. An organic-rich rock layer in some basins may thermally mature to generate mostly natural gas because of the dominance of higher plant debris contributing to its organic matter. Conversely, an organic-rich rock layer in other basins may thermally mature to generate mostly crude oil because of the dominance of lower plant debris contributing to its organic matter. More than one organic-rich rock layer may be deposited in the burial history of some basins with all, one, or none subsiding deep enough to thermally mature to generate petroleum. In other basins that have an organic-rich rock layer and sufficient burial to generate petroleum, the lack or scarcity of seals and reservoirs to collect generated petroleum may result in natural gas losses to the atmosphere or large degraded oil and tar deposits at or near the basin surface.
Research on these variables is critical to understanding the occurrences of known petroleum accumulations from which predictions can be made as to where undiscovered petroleum still resides within the Earth's crust. Research depends heavily on data collected from rock outcrops around and subsurface drilling in sedimentary basins. This geological data is essential to understanding of the development of sediment and rock layers (i.e., stratigraphy) within a basin and the history of their subsidence and trap development (i.e., tectonics). However, the vastness of sedimentary basins, limited well data, and migration of petroleum away from its source also requires research to 1) establish fingerprinting methods to determine genetic correlations among different petroleum types and their source and 2) conduct laboratory experiments to simulate petroleum generation and alteration to predict types, amounts, and extent of petroleum generated under varying subsurface conditions. Collectively, this understanding of genetically related petroleum, source rock identification, levels of thermal maturation, migration distances, and degrees of near-surface degradation allows construction of computer models of petroleum generation, migration, and accumulation through time within an evolving sedimentary basin. The USGS Energy Resources Team addresses these research issues under the Petroleum Processes Research Project.
*Figures are modified after those in Public Issues in Earth Sciences, USGS Circular 1115 entitled “The Future of Energy Gases” By P.J. McCabe, D.L. Gautier, M.D. Lewan, and C. Turner (1993).
Shale Gas
As natural gas demand increases in the United States, gas exploration across North America is increasingly focused on “unconventional” reservoirs, including shales (a broad term herein meant to include mudstone, claystone, and other fine-grained rocks). Although shales have been studied extensively in their role as source rocks (the rocks from which oil and gas generate they have only recently become... [+]
important reservoir rocks in North America, so research on them as it bears on their reservoir characteristics is extremely limited. Because North America is a mature petroleum province, shale gas (gas produced from shale reservoirs) is rapidly becoming an important domestic exploration target and an increasingly significant source of natural gas produced in the US and Canada. A systematic study of shale gas reservoirs is thus necessary in order to more fully appreciate the volume of contained petroleum resources and the mechanisms acting to create and preserve these reservoirs through geologic time. Such a study was recently launched by the USGS because a cursory look at some shale gas reservoirs in North America suggests that there are fundamental differences between them, making it difficult to generalize about these important reservoirs. Furthermore, there is little known about the role, if any, of clay minerals (Fig. 1A), the dominant mineral component of shale, in localizing organic material (the source of oil and/or gas) and their role in influencing the concentration of specific types of organic matter (Fig. 1B).
Figure 1A. Scanning electron photo showing clay
minerals (example shown by arrow), the
dominant mineral in shales.
Figure 1B. Scanning electron backscatter photo
showing the complex mixture within shales of
constituents including organic material, clays
I/S), fossil fragments, pyrite framboids, and
detrital quartz (Q). Other inorganic constituents
include carbonate (C) minerals.
Thus, scientists at the USGS are studying shales to address several fundamental questions concerning these important gas reservoirs such as, 1) what is the possible role of clay minerals in localizing and concentrating organic material in shale reservoirs? 2) what constitutes an effective shale gas source rock? and 3) can we define shale resources within a petroleum system framework? Addressing these questions will provide a greater understanding of the fundamental mechanisms responsible for shale gas accumulations and as such, construct a framework for future assessments of the undiscovered resources within these still enigmatic reservoirs.
Page Last Modified: Friday, October 05, 2012
|
|
Geochemistry Research Topics
Publication Search
A searchable database of thousands of published sources, dating back several decades
Find Data
USGS Energy Data Finder: Download GIS and tabular data, databases, geospatial web services (ArcGIS, WMS, KML)
EnergyVision
A single map viewer portal incorporating a range of maps, data and services
|