Abstract
Good pasture management practices favor effective use and recycling of nutrients. Nutrient cycles important in pasture systems are the water, carbon, nitrogen, and phosphorus cycles. This publication provides basic descriptions of these nutrient cycles then provides guidelines for managing pastures to enhance nutrient cycling efficiency for productive forage and livestock growth, soil health, and water quality.
Table of Contents
Introduction and Summary
As a pasture manager, what factors do you look at as indicators of high production and maximum profitability? You probably look at the population of animals stocked within the pasture. You probably look at vigor of plant regrowth. You probably also look at the diversity of plant species growing within the pasture and whether the plants are being grazed uniformly. But do you know how much water seeps into your soil or how much runs off the land into gullies or streams? Do you monitor how efficiently your plants are taking in carbon and forming new leaves, stems, and roots through photosynthesis? Do you know how effectively nitrogen and phosphorus are being used, cycled, and conserved on your farm? Are most of these nutrients being used for plant and animal growth? Or are they being leached into the groundwater or transported through runoff or erosion into lakes, rivers, and streams? If so, do you know how to change your pasture management practices to decrease these losses and increase the availability of these nutrients to your forages and animals?
Figure 1. Nutrient Cycles in Pastures |
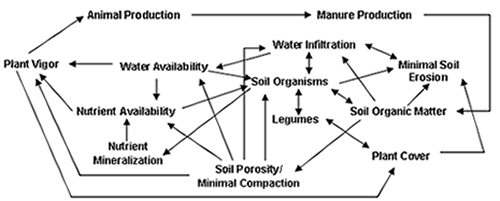 |
Healthy plant growth provides plant cover over the entire pasture. Cover from growing plants and plant residues protects the soil against erosion while returning organic matter to the soil. Organic matter provides food for soil organisms that mineralize nutrients from these materials and produce gels and other substances that enhance water infiltration and the capacity of soil to hold water and nutrients. |
Effective use and cycling of nutrients is critical for pasture productivity. As indicated in Figure 1 above, nutrient cycles are complex and interrelated. This document is designed to help you understand the unique components of water, carbon, nitrogen, and phosphorus cycles and how these cycles interact with one another. This information will help you monitor your pastures for breakdowns in nutrient cycling processes and then identify and implement productive pasture management practices to maximize effective nutrient cycling.
Water
Water is necessary for plant growth, for dissolving and transporting plant nutrients, and for the survival of soil organisms. Water can also be a destructive force, causing soil compaction, nutrient leaching, runoff, and erosion. Management practices that facilitate water movement into the soil and build the soil's capacity to store water will conserve water for plant growth and water recharge while minimizing its potential to cause nutrient losses. Water-conserving pasture management practices include:
- Minimizing soil compaction by not overgrazing pastures or using paddocks that have wet or saturated soils.
- Maintaining a complete coverage of forages and residues over all paddocks by not overgrazing pastures and by implementing practices that encourage animal movement across each paddock.
- Ensuring that forage plants include a diversity of grass and legume species with a variety of root systems capable of obtaining water and nutrients throughout the soil profile.
Carbon
Carbon is transformed from carbon dioxide into plant cell material through photosynthesis. It is the basic structural material for all cell life, and following the death and decomposition of cells it provides humus and other organic components that enhance soil quality. Plant nutrients such as nitrogen and phosphorus are chemically bound to carbon in organic materials. For these nutrients to become available for plant use, soil organisms need to break down the chemical bonds in a process called mineralization. If the amount of carbon compared to other nutrients is very high, more bonds will need to be broken and nutrient release will be slow. If the amount of carbon compared to other nutrients is low, fewer bonds will need to be broken and nutrient release will proceed relatively rapidly. Rapid nutrient release is preferred when plants are growing and are able to use the nutrients released. Slower nutrient release is preferred when plants are not actively growing (such as in the fall or winter) or if the amount of nutrients in the soil is already in excess of what plants can use. Pasture management practices that favor effective carbon use and cycling include:
- Maintaining a diversity of forage plants with a variety of leaf shapes and orientations to enhance photosynthesis and a variety of root growth habits to enhance nutrient uptake. A diversity of forages will provide balanced diet for grazing animals and soil organisms with a variety of food sources.
- Promoting healthy regrowth of forages by including a combination of grasses with both low and elevated growing points and by moving grazing animals frequently enough to minimize the removal of growing points.
- Maintaining a complete coverage of forages and residues over all paddocks to hold soil nutrients against runoff and leaching losses and ensure a continuous turnover of organic residues.
Nitrogen
Nitrogen is a central component of cell proteins and is used for seed production. It exists in several chemical forms and various microorganisms are involved in its transformations. Legumes, in association with specialized bacteria called rhizobia, as well as algae, are able to transform atmospheric nitrogen into a form available for plant use. Nitrogen in dead organic materials becomes available to plants through mineralization. Nitrogen can be lost from the pasture system through the physical processes of leaching, runoff, and erosion, the chemical process of volatilization, the biological process of denitrification, and through residue burning. Since nitrogen is needed in high concentration for forage production and it can be lost through a number of pathways, this nutrient is often the limiting factor in forage and crop production. Productive pasture management practices enhance the fixation and conservation of nitrogen while minimizing the potential for nitrogen losses. Practices that favor effective nitrogen use and cycling in pastures include:
- Maintaining stable or increasing percentages of legumes by not overgrazing pastures and minimizing nitrogen applications, especially in the spring.
- Protecting microbial communities involved in organic matter mineralization by minimizing practices that promote soil compaction and soil disturbance such as grazing wet soils, tillage, and cultivation.
- Incorporating manure and nitrogen fertilizers into the soil, and never applying these materials to saturated, snow-covered, or frozen soils.
- Avoiding pasture burning. If burning is required, it should be done very infrequently and then using a slow fire under controlled conditions.
- Applying fertilizers and manure according to a comprehensive nutrient management plan.
Phosphorus
Phosphorus is used for energy transformations within cells and is essential for plant growth. It is often the second most limiting mineral nutrient to plant production not only because it is critical for plant growth, but also because soil chemical bonds on soil particles hold the majority of phosphorus in forms not available for plant uptake. Phosphorus is also the major nutrient needed to stimulate the growth of algae in lakes and streams. Consequently, the inadvertent fertilization of these waterways with runoff water from fields and streams can cause degradation of water quality for drinking, recreational, or wildlife habitat uses. Regulations on the use of phosphorus-containing materials are becoming more widespread as society becomes increasingly aware of potential impacts agricultural practices have on water quality. Pasture management practices need to balance the need to ensure sufficient availability of phosphorus for plant growth with the need to minimize movement of phosphorus from fields to streams. Pasture management practices that protect this balance include:
- Minimizing the potential for compaction while providing organic inputs into the system to enhance activities of soil organisms and phosphorus mineralization.
- Incorporating manure and phosphorus fertilizers into the soil and never applying these materials to saturated, snow-covered, or frozen soils.
- Relying on soil tests, phosphorus index guidelines, and other nutrient management practices when applying fertilizers and manure to pastures.
Soil Life
Soil is a matrix of pore spaces filled with water and air, minerals, and organic matter. Although comprising only 1-6% of the soil, living organisms and decomposed organic materials are essential for providing plant nutrients, developing soil structure, holding water, and mediating nutrient transformations. Soil organic matter is composed of three components, stable humus, readily decomposable materials, and living organisms, also described as the very dead, the dead, and the living components of soil.(1)
Living organisms in soil include larger fauna such as moles and prairie dogs, macroorganisms such as insects and earthworms, and microorganisms including fungi, bacteria, yeasts, algae, protozoa, and nematodes. These living organisms break down the readily decomposable plant and animal material into nutrients, which become available for plant uptake. Organic matter residues from this decomposition process are subsequently broken down by other organisms until all that remains are complex compounds that are difficult to decompose. These complex end products of decomposition are known as humus.
Figure 2. Components of Soil Organic Matter |
 |
Soil contains 1-6% organic matter. Organic matter contains 3-9% active microorganisms. These organisms include plant life, bacteria and actinomycetes, fungi, yeasts, algae, protozoa, and nematodes. |
Humus, as well as fungal threads, bacterial gels, and earthworm feces form glues that hold soil particles together into forms, called aggregates, that give soil structure, enhance soil porosity, and allow water, air, and nutrients to flow through the soil. These residues of soil organisms also enhance nutrient and water holding capacity of soil. Lichen, algae, fungi, and bacteria form biological crusts over the soil surface. These crusts are important, especially in more arid rangelands, for enhancing water infiltration and providing nitrogen fixation.(2)
Maintaining effective nutrient cycling in the soil is highly dependent on maintaining an active and diverse population of soil organisms. Maintaining a substantial population of legumes in the pasture insures biological nitrogen fixation by bacteria associated with legume roots. Pasture management practices that favor populations of soil organisms by maintaining the soil as a habitat favorable for their growth and multiplication include:
- Maintaining a diversity of forages that promote a diverse population of soil organisms by providing them with a varied diet.
- Adding organic matter, such as forage residues and manure, to the soil to provide food for soil organism and facilitate the formation of aggregates to enhance soil porosity and the infiltration, flow, and drainage of water through the soil profile.
- Guarding against soil compaction, soil saturation, and the addition of amendments that might kill certain populations of soil organisms.
Back to top
Publication Overview
This publication is divided into five chapters:
The first chapter provides an overview of four nutrient cycles critical to plant production and water quality protection: the water, carbon, nitrogen, phosphorus, and sulfur cycles. Diagrams of each cycle are presented and the components of each cycle are explained, with emphasis on how these components are affected by pasture management practices. The description of each cycle concludes with a summary of pasture management practices to enhance efficient cycling of that nutrient.
The second chapter focuses on the effect of soil chemistry and mineralogy along with past and current land management practices on nutrient cycle transformations and nutrient availability. Management practice impacts discussed include soil compaction, organic matter additions and losses, soil pH, and method and timing of nutrient additions. The chapter concludes with a summary of pasture management practices for enhancing nutrient availability in pastures.
The third chapter discusses nutrient balances on grazed pastures and the availability of manure, residue, and fertilizer nutrients to forage growth. Factors affecting nutrient availability include nutrient content and consistency of manure, manure distribution as affected by paddock location and layout, and forage diversity. These factors, in turn, affect grazing intensity and pasture regrowth. A graph at the end of the chapter illustrates the interactions among these factors.
The fourth chapter describes the diversity of organisms involved in the decomposition of plant residues and manure in pastures. Included is a discussion of the impact of soil biological activity on the efficiency of nutrient cycles and forage production as well as impacts of pasture management on the activity of soil organisms. A soil health card developed for pastures provides a tool for qualitatively assessing the ability of soils to support healthy populations of soil organisms.
This publication concludes with a discussion of pasture management practices and their effects on water quality, soil erosion, water runoff, and water infiltration. Several topical water concerns are discussed: phosphorus runoff and eutrophication, nutrient and pathogen transport through subsurface drains, buffer management, and riparian grazing practices. A guide for assessing potential water quality impacts from pasture-management practices concludes this final chapter.
Back to top
Chapter 1: Nutrient Cycle Components, Interactions, and Transformations
The water, carbon, nitrogen, phosphorus, and sulfur cycles are the most important nutrient cycles operating in pasture systems. Each cycle has its complex set of interactions and transformations as well as interactions with the other cycles. The water cycle is essential for photosynthesis and the transport of nutrients to plant roots and through plant stems. It also provides the force and transport for nutrients lost through leaching runoff and erosion. The carbon cycle forms the basis for cell formation and soil quality. It initiates with photosynthesis and includes respiration, mineralization, immobilization, and humus formation. Atmospheric nitrogen is fixed into plant-available nitrogen by one type of bacteria, converted from the ammonical to nitrate form by another set of bacteria, and released back to the atmosphere by a fourth type of bacteria. A variety of soil organisms are involved in decomposition processes that release or mineralize nitrogen, phosphorus, sulfur, and other nutrients from plant residues and manure. Balances in the amount of these nutrients within organic materials, along with temperature and moisture conditions, determine which organisms are involved in the decomposition process and the rate at which this process proceeds.
Pasture management practices influence the interactions and transformations occurring within nutrient cycles. The efficiency of these cycles, in turn, influences the productivity of forage growth and the productivity of animals feeding on that forage. This chapter examines each of these cycles in detail and provides management guidelines for enhancing their efficiency.
Water Cycle
Water is critical for pasture productivity. It dissolves soil nutrients and moves them to plant roots. Inside plants, water and the dissolved nutrients support cell growth and photosynthesis. In the soil, water supports the growth and reproduction of insects and microorganisms that decompose organic matter. Water also can degrade pastures through runoff, erosion, and leaching, which cause nutrient loss and water pollution. Productive pastures are able to absorb and use water effectively for plant growth. Good pasture management practices promote water absorption by maintaining forage cover over the entire soil surface and by minimizing soil compaction by animals or equipment. Geology, soil type, and landscape orientation affect water absorption by soils and water movement through soils. Sloping land encourages water runoff and erosion; depressions and footslopes are often wet since water from upslope collects in these areas. Clay soils absorb water and nutrients, but since clay particles are very small, these soils compact easily. Sandy soils are porous and allow water to enter easily, but do not hold water and nutrients against leaching. Organic matter in soil absorbs water and nutrients, reduces soil compaction, and increases soil porosity. A relatively small increase in the amount of organic matter in soil can cause a large increase in the ability of soils to use water effectively to support plant production.
A forage cover over the entire paddock promotes water infiltration and minimizes soil compaction. |
Infiltration and water holding capacity
Water soaks into soils that have a plant or residue cover over the soil surface. This cover cushions the fall of raindrops and allows them to slowly soak into the soil. Roots create pores that increase the rate at which water can enter the soil. Long-lived, perennial bunch grass forms deep roots that facilitate water infiltration by conducting water into the soil.(3) Other plant characteristics that enhance water infiltration are significant litter production and large basal coverage.(4) In northern climates where snow provides a substantial portion of the annual water budget, maintaining taller grasses and shrubs that can trap and hold snow will enhance water infiltration.
Figure 3. Water Cycle
 |
The Water Cycle. Rain falling on soil can either be absorbed into the soil or be lost as it flows over the soil surface. Absorbed rain is used for the growth of plants and soil organisms, to transport nutrients to plant roots, or to recharge ground water. It can also leach nutrients through the soil profile, out of the reach of plant roots. Water flowing off the soil surface can transport dissolved nutrients as runoff or nutrients and other contaminates associated with sediments as erosion. |
Soils with a high water holding capacity absorb large amounts of water, minimizing the potential for runoff and erosion and storing water for use during droughts. Soils are able to absorb and hold water when they have a thick soil profile, contain a relatively high percentage of organic matter, and do not have a rocky or compacted soil layer, such as a hardpan or plowpan, close to the soil surface. An active population of soil organisms enhances the formation of aggregates and the formation of borrowing channels that provide pathways for water to flow into and through the soil. Management practices to enhance water infiltration and water holding capacity include:
- a complete coverage of forages and residues over the soil surface
- an accumulation of organic matter in and on the soil
- an active community of soil organisms involved in organic matter decomposition and aggregate formation
- water runoff and soil erosion prevention
- protection against soil compaction
Soil saturation
Soils become saturated when the amount of water entering exceeds the rate of absorption or drainage. A rocky or compacted lower soil layer will not allow water to drain or pass through, while a high water table will prevent water from draining through the profile. Water soaking into these soils is trapped or perched above the hard layer or high water table. Soils prone to saturation are usually located at the base of slopes, near waterways, or next to seeps.
Impact on crop production. Soil saturation affects plant production by exacerbating soil compaction, limiting air movement to roots, and ponding water and soil-borne disease organisms around plant roots and stems. Because soil pores are filled with water, roots and beneficial soil organisms lose access to air, which is necessary for their healthy growth. Soil compaction decreases the ability of air, water, nutrients, and roots to move through soils, even after soils have dried. Plants suffering from lack of air and nutrients are susceptible to disease attack since they are under stress, and wet conditions help disease organisms move from contaminated soil particles and plant residues to formerly healthy plant roots and stems.
Runoff and erosion potential. Soil saturation enhances the potential for runoff and erosion by preventing entry of additional water into the soil profile. Instead, excess water will run off the soil surface, often carrying soil and nutrients with it. Water can also flow horizontally under the surface of the soil until it reaches the banks of streams or lakes. This subsurface water flow carries nutrients away from roots, where they could be used for plant growth, and into streams or lakes where they promote the growth of algae and eutrophication.
Artificial drainage practices are often used on soils with a hardpan or a high water table to decrease the duration of soil saturation following rainfalls or snowmelts. This practice can increase water infiltration and decrease the potential for water runoff.(5) Unfortunately, most subsurface drains were installed before water pollution from agriculture became a concern and thus empty directly into drainage ways. Nutrients, pathogens, and other contaminants on the soil surface can move through large cracks or channels in the soil to drainage pipes where they are carried to surface water bodies.(6)
Soil compaction
Soil compaction occurs when animals or equipment move across soils that are wet or saturated, with moist soils being more easily compacted than saturated soils.(7) Compaction can also occur when animals or equipment continually move across a laneway or stand around watering tanks, headlands, or under shade. Animals trampling over the ground press down on soils, squeezing soil pore spaces together. Trampling also increases the potential for compaction by disturbing and killing vegetation.
Animals trampling over the ground press down on soils, squeezing soil pores together, which limits root growth and the movement of air, water, and dissolved nutrients. |
Soils not covered by forages or residues are easily compacted by the impact of raindrops. When raindrops fall on bare soil, their force causes fine soil particles to splash or disperse. These splash particles land on the soil surface, clog surface soil pores, and form a crust over the soil. Clayey soils are more easily compacted than sandy soils because clay particles are very small and sticky.
Compaction limits root growth and the movement of air, water, and dissolved nutrients through the soil. Compressing and clogging soil surface pores also decreases water infiltration and increases the potential for runoff. The formation of hardpans, plowpans, traffic pans, or other compacted layers within the soil decreases downward movement of water through the soil, causes rapid soil saturation and the inability of soils to absorb additional water. Compaction in pastures is remediated by root growth, aggregate formation, and activities of burrowing soil organisms. In colder climates, frost heaving is an important recovery process for compacted soils.(8)
Runoff and erosion
Runoff water dissolves nutrients and removes them from the pasture as it flows over the soil surface. Soil erosion transports nutrients and any contaminants, such as pesticides and pathogens, attached to soil particles. Because nutrient-rich clay and organic matter particles are small and lightweight, they are more readily picked up and moved by water than the nutrient-poor, but heavier sand particles. Besides depleting pastures of nutrients that could be used for forage production, runoff water and erosion carry nutrients and sediments that contaminate lakes, streams, and rivers.
Runoff water and erosion carry nutrients and sediments that contaminate lakes, streams, and rivers. |
Landscape conditions and management practices that favor runoff and erosion include sloping areas, minimal soil protection by forage or residues, intense rainfall, and saturated soils. While pasture managers should strive to maintain a complete forage cover over the soil surface, this is not feasible in practice due to plant growth habits and landscape characteristics. Plant residues from die-back and animal wastage during grazing provide a critical source of soil cover and soil organic matter. As mentioned above, forage type affects water infiltration and runoff. Forages with deep roots enhance water infiltration while plants with a wide vegetative coverage area or prostrate growth provide good protection against raindrop impact. Sod grasses that are short-lived and shallow rooted inhibit water infiltration and encourage water runoff. Grazing practices that produce clumps of forages separated by bare ground enhance runoff potential by producing pathways for water flow.
Evaporation and transpiration
Water in the soil profile can be lost through evaporation, which is favored by high temperatures and bare soils. Pasture soils with a thick cover of grass or other vegetation lose little water to evaporation since the soil is shaded and soil temperatures are decreased. While evaporation only affects the top few inches of pasture soils, transpiration can drain water from the entire soil profile. Transpiration is the loss of water taken up by plants through stomata in their leaves. Especially on sunny and breezy days, significant amounts of water can be absorbed from the soil by plant roots, taken up through the plant, and lost to the atmosphere through transpiration. A diversity of forage plants will decrease transpiration losses and increase water use efficiency due to differences among forage species in their ability to extract water from the soil and conserve it against transpiration.(9) Some invasive plant species, however, can deplete water stores through their high water usage.(4) Water not used for immediate plant uptake is held within the soil profile or is transported to groundwater reserves, which supply wells with water and decrease the impacts of drought.
Table 1. Water Cycle Monitoring |
If you answer no to all the questions, you have soils with high water-use efficiency. If you answered yes to some of the questions, water cycle efficiency of your soil will likely respond to improved pasture management practices. See Table 2, next page. |
|
YES |
NO |
Water infiltration / Water runoff |
1. Do patches of bare ground separate forage coverage? |
|
|
2. Are shallow-rooted sod grasses the predominant forage coverage? |
|
|
3. Can you see small waterways during heavy rainfalls or sudden snowmelts? |
|
|
4. Are rivulets and gullies present on the land? |
|
|
Soil saturation |
5. Following a rainfall, is soil muddy or are you able to squeeze water out of a handful of soil? |
|
|
6. Following a rainfall or snowmelt, does it take several days before the soil is no longer wet and muddy? |
|
|
7. Do forages turn yellow or die during wet weather? |
|
|
Soil compaction |
8. Do you graze animals on wet pasture? |
|
|
9. Are some soils in pasture bare, hard, and crusty? |
|
|
10. Do you have difficulty driving a post into (non-rocky) soils? |
|
|
Water retention / water evaporation and transpiration |
11. Do you have a monoculture of forages or are invasive species prominent components of the pasture? |
|
|
12. Do soils dry out quickly following a rainstorm? |
|
|
13. During a drought, do plants dry up quickly? |
|
|
Table 2: Pasture Management Practices for Efficient Water Cycling in Pastures |
Ensure forage and residue coverage across the entire pasture
- Use practices that encourage animal movement throughout the pasture and discourage congregation in feeding and lounging areas
- Use practices that encourage regrowth of forage plants and discourage overgrazing
- Use a variety of forages with a diversity of root systems and growth characteristics
Pasture management during wet weather
- Use well-drained pastures or a "sacrificial pasture" that is far from waterways or water bodies
- Avoid driving machinery on pastures that are wet or saturated
- Avoid spreading manure or applying fertilizers
Artificial drainage practices
- Avoid grazing animals on artificially drained fields when drains are flowing
- Avoid spreading manure or applying fertilizers when drains are flowing
- Ensure that drains empty into a filter area or wetland rather than directly into a stream or drainage way.
|
Back to top
Carbon Cycle
Effective carbon cycling in pastures depends on a diversity of plants and healthy populations of soil organisms. Plants form carbon and water into carbohydrates through photosynthesis. Plants are most able to conduct photosynthesis when they can efficiently capture solar energy while also having adequate access to water, nutrients, and air. Animals obtain carbohydrates formed by plants when they graze on pastures or eat hay or grains harvested from fields. Some of the carbon and energy in plant carbohydrates is incorporated into animal cells. Some of the carbon is lost to the atmosphere as carbon dioxide, while energy is lost as heat during digestion and as the animal grows and breathes.
Humus maintains soil tilth and enhances water and nutrient absorbtion. |
Carbohydrates and other nutrients not used by animals are returned to the soil in the form of urine and manure. These organic materials provide soil organisms with nutrients and energy. As soil organisms use and decompose organic materials, they release nutrients from these materials into the soil. Plants then use the released, inorganic forms of nutrients for their growth and reproduction. Soil organisms also use nutrients from organic materials to produce substances that bind soil particles into aggregates. Residues of organic matter that resist further decomposition by soil organisms form soil humus. This stable organic material is critical for maintaining soil tilth and enhancing the ability of soils to absorb and hold water and nutrients.
Carbohydrate formation
For productive growth, plants need to effectively capture solar energy, absorb carbon dioxide, and take up water from the soil to produce carbohydrates through photosynthesis. In pastures, a combination of both broadleaf plants and grasses allows for efficient capture of solar energy by a diversity of leaf shapes and leaf angles. Taller plants with more erect leaves capture light, even at the extreme angles of sunrise and sunset. Horizontal leaves capture the sun at midday or when it is more overhead.
Two methods for transforming carbon into carbohydrates through photosynthesis are represented in diversified pastures. Broadleaf plants and cool season grass have a pathway that is efficient in the production of carbohydrates but is sensitive to dry conditions. Warm season grasses have a pathway that is more effective in producing carbohydrates during hot, summer conditions. A combination of plants representing these two pathways ensures effective forage growth throughout the growing season. A diversity of root structures also promotes photosynthesis by giving plants access to water and nutrients throughout the soil profile.
Figure 4. Carbon Cycle
|
The Carbon Cycle begins with plants taking up carbon dioxide from the atmosphere in the process of photosynthesis. Some plants are eaten by grazing animals, which return organic carbon to the soil as manure, and carbon dioxide to the atmosphere. Easily broken-down forms of carbon in manure and plant cells are released as carbon dioxide when decomposing soil organisms respire. Forms of carbon that are difficult to break down become stabilized in the soil as humus. |
Organic matter decomposition
Pasture soils gain organic matter from growth and die-back of pasture plants, from forage wastage during grazing, and from manure deposition. In addition to the recycling of above ground plant parts, every year 20-50% of plant root mass dies and is returned to the soil system. Some pasture management practices also involve the regular addition of manure from grazing animals housed during the winter or from poultry, hog, or other associated livestock facilities.
A healthy and diverse population of soil organisms is necessary for organic matter decomposition, nutrient mineralization, and the formation of soil aggregates. |
A healthy and diverse population of soil organisms is necessary for organic matter decomposition, nutrient mineralization, and the formation of soil aggregates. Species representing almost every type of soil organism have roles in the break down of manure, plant residues, and dead organisms. As they use these substances for food and energy sources, they break down complex carbohydrates and proteins into simpler chemical forms. For example, soil organisms break down proteins into carbon dioxide, water, ammonium, phosphate, and sulfate. Plants require nutrients to be in this simpler, decomposed form before they can use them for their growth.
To effectively decompose organic matter, soil organisms require access to air, water, and nutrients. Soil compaction and saturation limit the growth of beneficial organisms and promote the growth of anaerobic organisms, which are inefficient in the decomposition of organic matter. These organisms also transform some nutrients into forms that are less available or unavailable to plants. Nutrient availability and nutrient balances in the soil solution also affect the growth and diversity of soil organisms. To decompose organic matter that contains a high amount of carbon and insufficient amounts of other nutrients, soil organisms must mix soil solution nutrients with this material to achieve a balanced diet.
Balances between the amount of carbon and nitrogen (C:N ratio) and the amount of carbon and sulfur (C:S ratio) determine whether soil organisms will release or immobilize nutrients when they decompose organic matter. Immobilization refers to soil microorganisms taking nutrients from the soil solution to use in the decomposition process of nutrient-poor materials. Since these nutrients are within the bodies of soil organisms, they are temporarily unavailable to plants. In soils with low nutrient content, this can significantly inhibit plant growth. However, immobilization can be beneficial in soils with excess nutrients. This process conserves nutrients in bodies of soil organisms, where they are less likely to be lost through leaching and runoff.(10)
In soils with low nutrient content, nutrient immobilization inhibits plant growth. In soils with excess nutrients, immobilization conserves nutrients in the bodies of soil organisms, where they are less likely to be lost through leaching and runoff. |
Populations of soil organisms are enhanced by soil that is not compacted and has adequate air and moisture, and by additions of fresh residues they can readily decompose. Soil-applied pesticides can kill many beneficial soil organisms, as will some chemical fertilizers. Anhydrous ammonia and fertilizers with a high chloride content, such as potash, are particularly detrimental to soil organism populations. Moderate organic or synthetic fertilizer additions, however, enhance populations of soil organisms in soils with low fertility.
Soil humus and soil aggregates
Besides decomposing organic materials, bacteria and fungi in the soil form gels and threads that bind soil particles together. These bound particles are called soil aggregates. Worms, beetles, ants, and other soil organisms move partially decomposed organic matter through the soil or mix it with soil in their gut, coating soil particles with organic gels. As soil particles become aggregated, soil pore size increases and soils become resistant to compaction. The organic compounds that hold aggregates together also increase the ability of soils to absorb and hold water and nutrients.
As manure and plant residues are decomposed by soil organisms, carbon dioxide is released as respiration and waste materials are produced, which are further decomposed by other soil organisms. Because carbon is lost to respiration at each stage of this decomposition process, the remaining material increases in nitrogen content. The remaining material also increases in chemical complexity and requires increasingly specialized species of decomposers. Efficient decomposition of organic matter thus requires a diversity of soil organisms. Humus is the final, stable product of decomposition, formed when organic matter is only broken down by soil organisms slowly or with difficulty. Humus-coated soil particles form aggregates that are soft, crumbly, and somewhat greasy-feeling when rubbed together.
Table 3: Typical C:N, C:S, and N:S Ratios |
Typical C:N, C:S and N:S ratios of plant residues, excetera of ruminant animals and biomass of soil microorganisms decomposing in grassland soils (based on values for % in dry matter) |
|
%N |
C:N |
%S |
C:S |
N:S |
Dead grass |
1.8 |
26.6:1 |
0.15 |
320:1 |
12:1 |
Dead clover |
2.7 |
17.7:1 |
0.18 |
270:1 |
15:1 |
Grass roots |
1.4 |
35:1 |
0.15 |
330:1 |
9:1 |
Clover roots |
3.8 |
13.2:1 |
0.35 |
140:1 |
10:1 |
Cattle feces |
2.4 |
20:1 |
0.30 |
160:1 |
8:1 |
Cattle urine |
11.0 |
3.9:1 |
0.65 |
66:1 |
17:1 |
Bacteria |
15.0 |
3.3:1 |
1.10 |
45:1 |
14:1 |
Fungi |
3.4 |
12.9:1 |
0.40 |
110:1 |
8.5:1 |
from Whitehead, 2000 (11) |
Preventing organic matter losses
Perennial plant cover in pastures not only provides organic matter inputs, it also protects against losses of organic matter through erosion. Soil coverage by forages and residues protects the soil from raindrop impact while dense root systems of forages hold the soil against erosion while enhancing water infiltration. Fine root hairs also promote soil aggregation. In addition, a dense forage cover shades and cools the soil. High temperatures promote mineralization and loss of organic matter, while cooler temperatures promote the continued storage of this material within the plant residues and the bodies of soil organisms.
Table 4: Pature Managment Practices for Efficient Carbon Cycling
Ensure forage and residue coverage and manure deposition across the entire pasture
- Use practices that encourage animal movement throughout the pasture and discourage congregation in feeding and lounging areas
Promote healthy forage growth and recovery following grazing
- Use a variety of forages with a diversity of leaf types and orientations
- Use a combination cool and warm season forages with a diversity of shoot and root growth characteristics
- Conserve sufficient forage leaf area for efficient plant regrowth by monitoring pastures and moving grazing animals to another pasture in a timely manner
- Maintain soil tilth for healthy root growth and nutrient uptake
Encourage organic matter decomposition by soil organisms
- Use management practices that minimize soil compaction and soil erosion
- Minimize use of tillage and cultivation practices
- Maintain a diversity of forage species to provide a variety of food sources and habitats for a diversity of soil organisms
- Avoid the use of soil applied pesticides and concentrated fertilizers that may kill or inhibit the growth of soil organisms
Encourage soil humus and aggregate formation
- Include forages with fine, branching root systems to promote aggregate formation
- Maintain organic matter inputs into the soil to encourage the growth of soil organisms
- Maintain coverage of forages and plant residues over the entire paddock to provide organic matter additions and discourage its rapid degradation.
|
Back to top
Nitrogen Cycle
Nitrogen is a primary plant nutrient and a major component of the atmosphere. In a pasture ecosystem, almost all nitrogen is organically bound. Of this, only about 3% exists as part of living plant, animal, or microbial cells while the remainder is a component of decomposed organic matter or humus. A very small percent of the total nitrogen (<0.01%) exists as plant available nitrogen in the form of ammonium or nitrate.(12)
Nitrogen becomes available for the growth of crop plants and soil organisms through nitrogen fixation, nitrogen fertilizer applications, the return of manure to the land, and through the mineralization of organic matter in the soil. Nitrogen fixation occurs mainly in the roots of legumes that form a symbiotic association with a bacteria species called rhizobia. Some algae and free-living bacteria are also able to transform atmospheric nitrogen into a form available for plant growth. Fertilizer factories use a combination of high pressure and high heat to combine atmospheric nitrogen and hydrogen into nitrogen fertilizers. Animals deposit organically-bound nitrogen in feces and urine. Well-managed pastures accumulate stores of organic matter in the soil and in plant residues. Decomposition and mineralization of nutrients in these materials can provide significant amounts of nitrogen to plants and other organisms in the pasture system.
Figure 5. Nitrogen Cycle
|
The Nitrogen Cycle. Nitrogen enters the cycle when atmospheric nitrogen is fixed by bacteria. Nitrogen in the ammonical form is transformed into nitrite and nitrate by bacteria. Plants can use either ammonia or nitrate for growth. Nitrogen in plant cells can be consumed by animals and returned to the soil as feces or urine. When plants die, soil organisms decompose nitrogen in plant cells and release it as ammonia. Nitrate nitrogen can be lost through the physical process of leaching or through the microbially-mediated process of denitrification. Nitrogen in the ammonical form can be lost to the atmosphere in the chemical process of volatilization. |
Plants use nitrogen for the formation of proteins and genetic material. Grazing animals that consume these plants use some of the nitrogen for their own growth and reproduction; the remainder is returned to the earth as urine or manure. Soil organisms decompose manure, plant residues, dead animals, and microorganisms, transforming nitrogen-containing compounds in their bodies into forms are available for use by plants.
Nitrogen is often lacking in pasture systems since forage requirements for this nutrient are high and also because it is easily lost to the environment. Nitrogen is lost from pasture systems through microbiological, chemical, and physical processes. Dry followed by wet weather provides optimal conditions for bacteria to transform nitrogen from plant-available forms into atmospheric nitrogen through denitrification. Chemical processes also transform plant-available nitrogen into atmospheric nitrogen through volatilization. In pastures, this often occurs after manure or nitrogen fertilizers are applied to the soil surface, especially during warm weather. Physical processes are involved in the downward movement of nitrogen through the soil profile during leaching.
Nitrogen fixation
Plants in the legume family, including alfalfa, clover, lupines, lespedeza, and soybeans, form a relationship with a specialized form of bacteria called rhizobia. These bacteria have the ability to fix or transform atmospheric nitrogen into a form of nitrogen plants can use for their growth. Rhizobia form little balls or nodules on the roots of legumes. If these balls are white or pinkish on the inside, they are actively fixing nitrogen. Nodules that are grey or black inside are dead or no longer active. Legume seeds should be dusted with inoculum (a liquid goo or powder containing the appropriate type of rhizobia) prior to planting to ensure that the plant develops many nodules and has maximal ability to fix nitrogen. Some algae and other microorganisms that live in the soil are also able to fix and provide nitrogen to plants.
Table 5: Nitrogen Fixation by Legumes |
|
#N/acre/year |
Alfalfa |
150-350 |
White clover |
112-190 |
Hairy vetch |
110-168 |
Red clover |
60-200 |
Soybeans |
35-150 |
Annual lespedeza |
50-193 |
Birdsfoot trefoil |
30-130 |
From Joost, 1996, (13) |
Legumes require higher amounts of phosphorus, sulfur, boron, and molybdenum than non-legumes to form nodules and fix nitrogen. If these nutrients are not available in sufficient amounts, nitrogen fixation will be suppressed. When nitrogen levels in the soil are high due to applications of manure or nitrogen fertilizers, nitrogen fixation by legumes also decreases because nitrogen fixation requires more energy than does root uptake of soluble soil nitrogen. Nitrogen fixed by legumes and rhizobia is available primarily to the legumes while they are growing. When pasture legume nodules, root hairs, and above-ground plant material dies and decomposes, nitrogen in this material can become available to pasture grasses.(14)
However, while legumes are still growing, mycorrhizal fungi can form a bridge between the root hairs of legumes and nearby grasses. This bridge facilitates the transport of fixed nitrogen from legumes to linked grasses. Depending on the nitrogen content of the soil and the mix of legumes and grasses in a pasture, legumes can transfer between 20-40% of their fixed nitrogen to grasses during the growing season.(15) A pasture composed of at least 20-45% legumes (dry matter basis) can meet and sustain the nitrogen needs of the other forage plants in the pasture.(16)
Legumes can transfer up to 40% of their fixed nitrogen to grasses during the growing season. |
Grazing management affects nitrogen fixation through the removal of herbage, deposition of urine and manure, and induced changes in moisture and temperature conditions in this soil. Removal of legume leaf area decreases nitrogen fixation by decreasing photosynthesis and plant competitiveness with grasses. Urine depositions decrease nitrogen fixation by adjacent plants since it creates an area of high soluble nitrogen availability. Increases in moisture in compacted soils or temperature in bare soils will also decrease nitrogen fixation since rhizobia are sensitive to wet and hot conditions.
Nitrogen mineralization
Decomposition of manure, plant residues or soil organic matter by organisms in the soil results in the formation of ammonical nitrogen. Protozoa, amoebae and nematodes are prolific nitrogen mineralizers, cycling 14 times their biomass each year. While bacteria only cycle 0.6 times their biomass, because of their large numbers in soil they produce a greater overall contribution to the pool of mineralized nitrogen.(17) Plants can use ammonical nitrogen for their growth, but under aerobic conditions two types of bacteria usually work together to rapidly transform ammonia first into nitrite and then into nitrate before it is used by plants.
Mineralized nitrogen is a very important source of nitrogen in most grasslands. As discussed in Carbon Cycle, above, efficient decomposition and release of nitrogen from decomposing residues depends on residues containing a ratio of carbon to nitrogen in balance with the nutrient needs of decomposing organisms. If the nitrogen content of residues is insufficient, soil organisms will extract nitrogen from the soil solution to satisfy their nutrient needs.
Nitrogen losses to the atmosphere
Under wet or anaerobic conditions, bacteria transform nitrate nitrogen into atmospheric nitrogen. This process, called denitrification, reduces the availability of nitrogen for plant use. Denitrification occurs when dry soil containing nitrate becomes wet or flooded and at the edges of streams or wetlands where dry soils are adjacent to wet soils.
Volatilization is the transformation of ammonia into atmospheric nitrogen. This chemical process occurs when temperatures are high and ammonia is exposed to the air. Incorporation of manure or ammonical fertilizer into the soil decreases the potential for volatilization. In general, 5-25% of the nitrogen in urine is volatilized from pastures.(11) A thick forage cover and rapid manure decomposition can reduce volatilization from manure.
Nitrogen leaching
Soil particles and humus are unable to hold nitrate nitrogen very tightly. Water from rainfall or snowmelt readily leaches soil nitrate downward through the profile, putting it out of reach of plant roots or moving it into the groundwater. Leaching losses are greatest when the water table is high, the soil sandy or porous, or when rainfall or snowmelt is severe. In pastures, probably the most important source of nitrate leaching is from urine patches.(18) Cattle urine typically leaches to the depth of 16 inches while sheep urination only leaches six inches into the ground.(19) Leaching may also be associated with the death of legume nodules during dry conditions.(20) Methods for reducing the potential for nitrate leaching include maintaining an actively growing plant cover over the soil surface, coordinating nitrogen applications with the period of early plant growth, not applying excess nitrogen to soils, and encouraging animal movement and distribution of manure across paddocks. Actively growing plant roots take up nitrate from the soil and prevent it from leaching. If the amount of nitrogen applied to the soil is in excess of what plants need or is applied when plants are not actively growing, nitrate not held by plants can leach through the soil. Spring additions of nitrogen to well-managed pastures can cause excessive plant growth and increase the potential for nitrogen leaching since significant amounts of nitrogen are also being mineralized from soil organic matter as warmer temperatures increase the activity of soil organisms.
Nitrate levels in excess of 10 ppm in drinking water can cause health problems for human infants, infant chickens and pigs, and both infant and adult sheep, cattle, and horses.(21) Pasture forages can also accumulate nitrate levels high enough to cause health problems. Conditions conducive for nitrate accumulation by plants include acid soils, low molybdenum, sulfur, and phosphorus content, soil temperatures lower than 55° F, and good soil aeration.(22)
This nitrate toxicity health problem is called methemoglobinemia, the common name being "blue baby syndrome" when seen in human babies. In this syndrome, nitrate binds to hemoglobin in the blood, reducing the blood's ability to carry oxygen through the body. Symptoms in human infants and young animals include difficulty breathing. Pregnant animals that recover may abort within a few days. Personnel from the Department of Health can test wells to determine whether nitrate levels are dangerously high.
Table 6: Estimated Nitrogen Balance (pounds/acre) for Two Grassland Managment Systems |
|
Moderately managed grass-clover |
Extensively grazed grass |
Inputs |
Nitrogen fixation |
134 |
9 |
Atmospheric deposition |
34 |
19 |
Fertilizer |
0 |
0 |
Supplemental feed |
0 |
0 |
Recycled nutrients |
Uptake to herbage |
270 |
67 |
Herbage consumption by animals |
180 |
34 |
Dead herbage to soil |
90 |
34 |
Dead roots to soil |
56 |
34 |
Manure to soil |
134 |
28 |
Outputs |
Animal weight gain |
28 |
4 |
Leaching/runoff/erosion |
56 |
6 |
Volitalization |
17 |
3 |
Denitrification |
22 |
2 |
Gain to soil |
56 |
13 |
From: Whitehead, 2000 (11) |
Nitrogen loss through runoff and erosion
Runoff and erosion caused by rainwater or snowmelt can transport nitrogen on the soil surface. Erosion removes soil particles and organic matter that contain nitrogen; runoff transports dissolved ammonia and nitrate. Incorporation of manure and fertilizers into the soil reduces the exposure of these nitrogen sources to rainfall or snowmelt, thus reducing the potential for erosion. In pasture systems, however, incorporation is usually impractical and can increase the potential for erosion. Instead, a complete cover of forages and plant residues should be maintained over the soil surface to minimize raindrop impact on the soil, enhance water infiltration, help trap sediments and manure particles, and reduce the potential for runoff and erosion. A healthy and diverse population of soil organisms, including earthworms and dung beetles that rapidly incorporate manure nitrogen into the soil and into their cells can further reduce the risk of nitrogen runoff from manure. Since increased water infiltration decreases the potential for runoff but increases the potential for leaching, risks of nitrate losses from runoff need to be balanced against leaching risks.
Table 7: Pasture Management Practices for Efficient Nitrogen Cycling
Ensure effective nitrogen fixation by legumes
- Ensure that phosphorus, sulfur, boron, and molybdenum in the soil are sufficient for effective nitrogen fixation
- Apply inoculum to legume seeds when sowing new pastures to ensure nodulation of legume roots
- Ensure that legumes represent at least 30% of the forage cover
- Maintain stable or increasing percentages of legumes to grasses and other non-legumes in pastures over time
- Establish forages so that legumes and grasses grow close to one another to allow for the transfer of nitrogen from legumes to grasses
Encourage nitrogen mineralization by soil organisms
- Use management practices that minimize soil compaction and soil erosion
- Minimize use of tillage and cultivation practices
- Maintain a diversity of forage species to provide a variety of food sources and habitats for a diversity of soil organisms
- Use grazing management practices that encourage productive forage growth and that return and maintain residues within paddocks
- Avoid application of sawdust, straw, or other high-carbon materials unless these materials are mixed with manure or composted prior to application
- Avoid the use of soil applied pesticides and concentrated fertilizers that may kill or inhibit the growth of soil organisms
Avoid nitrogen losses
- Minimize nitrogen volatilization by avoiding surface application of manure, especially when the temperature is hot or there is minimal forage cover over the soil
- Minimize nitrogen leaching by not applying nitrogen fertilizer or manure when soil is wet or just prior to rainstorms and by encouraging animal movement and distribution of urine spots across paddocks
- Minimize nitrogen leaching by not applying nitrogen fertilizer or manure to sandy soils except during the growing season
- Rely on mineralization of organic residues to supply most or all of your forage nitrogen needs in the spring. Minimize the potential for nitrogen leaching by limiting spring applications of nitrogen
- Minimize nitrogen losses caused by erosion by using management practices that maintain a complete cover of forages and residues over the pasture surface
Ensure effective use of nitrogen inputs
- Use management practices that encourage the even distribution of manure and urine across paddocks
- Rely on soil tests and other nutrient management practices when applying fertilizers and manure to pastures
|
Back to top
Phosphorus Cycle
Like nitrogen, phosphorus is a primary plant nutrient. Unlike nitrogen, phosphorus is not part of the atmosphere. Instead, it is found in rocks, minerals, and organic matter in the soil. The mineral forms of phosphorus are apatitite, which may be in a carbonate, hydroxide, fluoride, or chloride form, and iron or aluminum phosphates. These minerals are usually associated with basalt and shale rocks. Chemical reactions and microbial activity affect the availability of phosphorus for plant uptake. Under acid conditions, phosphorus is held tightly by aluminum and iron in soil minerals. Under alkaline conditions, phosphorus is held tightly by soil calcium.
Plants use phosphorus for energy transfer and reproduction. Legumes require phosphorus for effective nitrogen fixation. Animals consume phosphorus when they eat forages. Phosphorus not used for animal growth is returned to the soil in manure. Following decomposition by soil organisms, phosphorus again becomes available for plant uptake.
Figure 6. Phosphorus Cycle
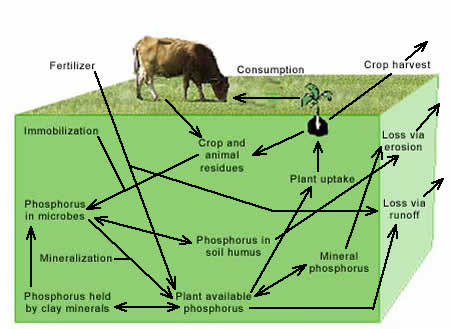
|
The phosphorus cycle is affected by microbial and chemical transformations. Soil organisms mineralize or release phosphorus from organic matter. Phosphorus is chemically bound to iron and aluminum in acid soils while it is bound to calcium in alkaline soils. Soil-bound phosphorus can be lost due to erosion while runoff waters can transport soluble phosphorus found at the soil surface. |
Mycorrhizae
Mycorrhizal fungi attach to plant roots and form thin threads that grow through the soil and wrap around soil particles. These thin threads increase the ability of plants to obtain phosphorus and water from soils. Mycorrhizae are especially important in acid and sandy soils where phosphorus is either chemically bound or has limited availability. Besides transferring phosphorus and water from the soil solution to plant roots, mycorrhizae also facilitate the transfer of nitrogen from legumes to grasses. Well-aerated and porous soils, and soil organic matter, favor mycorrhizal growth.
Soil chemistry and phosphorus availability
Phosphorus is tightly bound chemically in highly weathered acid soils that contain high concentrations of iron and aluminum. Active calcium in neutral to alkaline soils also forms tight bonds with phosphorus. Liming acid soils and applying organic matter to either acid or alkaline soils can increase phosphorus availability. In most grasslands, the highest concentration of phosphorus is in the surface soils associated with decomposing manure and plant residues.
Phosphorus loss through runoff and erosion
Unlike nitrogen, phosphorus is held by soil particles. It is not subject to leaching unless soil levels are excessive. However, phosphorus can move through cracks and channels in the soil to artificial drainage systems, which can transport it to outlets near lakes and streams. Depending on the soil type and the amount of phosphorus already in the soil, phosphorus added as fertilizer or manure may be readily lost from fields and transported to rivers and streams through runoff and erosion. The potential for phosphorus loss through runoff or erosion is greatest when rainfall or snowmelt occurs within a few days following surface applications of manure or phosphorus fertilizers.
Continuous manure additions increase the potential for phosphorus loss from the soil and the contamination of lakes and streams. This is especially true if outside manure sources are used to meet crop or forage nutrient needs for nitrogen. The ratio of nitrogen to phosphate in swine or poultry manure is approximately 1:1 while the ratio of the ratio of nitrogen to phosphate taken up by forage grasses is between 2.5-3.8:1. Thus, manure applied for nitrogen requirements will provide 2.5-3.8 times the amount of phosphorus needed by plants.(23) While much of this phosphorus will be bound by chemical bonds in the soil and in the microbial biomass, continuous additions will exceed the ability of the soil to store excess phosphorus and the amount of soluble phosphorus, the form available for loss by runoff, will increase. To decrease the potential for phosphorus runoff from barnyard manure or poultry litter, alum, or aluminum oxide, can be added to bind phosphorus in the manure.(24)
Supplemental feeds are another source of phosphorus inputs into grazing systems, especially for dairy herds. Feeds high in phosphorus increase the amount of phosphorus deposited on pastures as manure. To prevent buildup of excess phosphorus in the soil, minimize feeding of unneeded supplements, conduct regular soil tests on each paddock, and increase nutrient removals from high or excessively fertile paddocks through haying.
Phosphorus index guidelines consider:
- the amount of phosphorus in the soil
- manure and fertilizer application rates, methods, and timing
- runoff and erosion potential
- distance from a water body.
|
Phosphorus runoff from farming operations can promote unwanted growth of algae in lakes and slow-moving streams. Regulations and nutrient management guidelines are being developed to decrease the potential for phosphorus movement from farms and thus reduce risks of lake eutrophication. Land and animal management guidelines, called "phosphorus indices," are being developed across the U.S. to provide farmers with guidelines for reducing "non-point" phosphorus pollution from farms.(25) These guidelines identify risk factors for phosphorus transport from fields to water bodies based on the concentration of phosphorus in the soil, timing and method of fertilizer and manure applications, potential for runoff and erosion, and distance of the field from a water body.(26)
Although the total amount of phosphorus lost from fields is greatest during heavy rainstorms, snowmelts, and other high runoff occurrences, relatively small amounts of phosphorus running off from fields into streams at low water level in summer pose a higher risk for eutrophication. This is because phosphorus is more concentrated at low flows than during high flow periods.(27) Conditions for concentrated flows of phosphorus into low-flow streams include location near streams of barnyards or other holding areas without runoff containment or filtering system, extensive grazing of animals near streams without riparian buffers, and animal access to streams.
Table 8: Pasture Management Practices for Efficient Phosphorus Cycling
Encourage phosphorus mineralization by soil organisms
- Use management practices that minimize soil compaction and soil erosion
- Minimize use of tillage and other cultivation practices
- Maintain a diversity of forage species to provide a variety of food sources and habitats for a diversity of soil organisms
- Avoid application of sawdust, straw, or other high-carbon materials unless these materials are mixed with manure or composted prior to application
- Avoid the use of soil-applied pesticides and concentrated fertilizers that may kill or inhibit the growth of soil organisms
Avoid phosphorus losses
- Minimize phosphorus losses caused by erosion by using management practices that maintain a complete cover of forages and residues over the pasture surface
- Minimize phosphorus losses caused by runoff by not surface-applying fertilizer or manure to soil that is saturated, snow-covered, or frozen
- Avoid extensive grazing of animals in or near streams especially when land is wet or saturated or when streams are at low flow
Ensure effective use of phosphorus inputs
- Use management practices that encourage the even distribution of manure and urine across paddocks
- Rely on soil tests, phosphorus index guidelines, and other nutrient management practices when applying fertilizers and manure to pastures
|
Back to top
Secondary Nutrients
Potassium and the secondary nutrients, calcium, magnesium, and sulfur, play a critical role in plant growth and animal production. Potassium, calcium, and magnesium are components of clay minerals. The soil parent-material prmarily influences the availability of these plant nutrients. For example, soils derived from granite contain, on the average, nine times more potassium than soils derived from basalt, while soils derived from limestone have half the amount. Conversely, soils derived from limestone have, on the average, four times more calcium than soils derived from basalt and thirty times more than soils derived from granite.(11)
Potassium
Potassium, like all plant nutrients, is recycled through plant uptake, animal consumption, and manure deposition. The majority of potassium is found in urine. Potassium levels can become excessive in fields that have received repeated, high applications of manure. Application of fertilizer nitrogen increases the potassium uptake by grasses if the soil has an adequate supply of potassium. Consumption of forages that contain more than 2% potassium can cause problems in breeding dairy cattle and in their recovery following freshening.(28) High potassium levels, especially in lush spring forage, can cause nutrient imbalance resulting in grass tetany.
Calcium and magnesium
Calcium and magnesium are components of liming materials used to increase soil pH and reduce soil acidity. However, the use of lime can also be important for increasing the amount of calcium in the soil or managing the balance between calcium and magnesium. Increasing the calcium concentration in the soil may enhance biological activity in the soil.(29) Managing this balance is especially important for decreasing the tendency for grass tetany, a nutritional disorder of ruminants caused by low levels of magnesium in the diet. Magnesium may be present in the soil in sufficient amounts for plant growth, but its concentration may be out of balance with the nutrient needs of plants and animals. When calcium and potassium have a high concentration in the soil compared to magnesium, they will limit the ability of plants to take up magnesium. Under these conditions, the magnesium concentration needs to be increased relative to calcium. Dolomite lime, which contains magnesium carbonate, can be used to both lime soils and increase the availability of magnesium. Phosphorus fertilization of tall fescue in Missouri was also shown to increase the availability of magnesium sufficiently to decrease the incidence of grass tetany in cattle.(30) This result was probably due to the stimulation of grass growth during the cool wet spring conditions that are conducive to the occurrence of grass tetany.
Phosphorus fertilization of tall fescue decreased the incidence of grass tetany in cattle since it stimulated grass growth and increased the availability of magnesium during cool wet spring conditions. |
Sulfur
Sulfur increases the protein content of pasture grasses and increases forage digestibility and effectiveness of nitrogen use.(31) In nature, sulfur is contained in igneous rocks, such as granite and basalt, and is a component of organic matter. In areas downwind from large industrial and urban centers, sulfur contributions from the atmosphere in the form of acid rain can be considerable. Fertilizer applications of nitrogen as ammonium sulfate or as sulfur-coated urea also contribute to sulfur concentration in soils. However, as environmental controls for acid rain improve, other sources of nitrogen fertilizer are used, and forage production increases, pasture needs for sulfur fertilization increase.
As a component of organic matter, microbial processes affect sulfur availability. Like nitrogen, the sulfur content of organic matters determines whether nutrients will be mineralized or immobilized. Also, similar to nitrogen, the sulfur content of grasses decreases as they become older and less succulent. Thus, soil organisms will decompose younger plants more rapidly and release nutrients from this organic matter while they will decompose older plant material more slowly and may immobilize soil nutrients in the process of decomposition.
Chemical and biological processes are involved in sulfur transformations. In dry soils that become wet or waterlogged, chemical processes transform sulfur from the sulfate to sulfide form. If these wet soils dry out or are drained, bacteria transform sulfide to sulfate. Similar to nitrate, sulfate is not readily absorbed by soil minerals, especially in soils with a slightly acid to neutral pH. As a result, sulfate can readily leach through soils that are sandy or highly permeable.
Table 9: Pasture Management Practices for Efficient Cycling of Potassium, Calcium, Magnesium, and Sulfur
Encourage nutrient mineralization by soil organisms
- Use management practices that minimize soil compaction and soil erosion
- Minimize use of tillage and other cultivation practices
- Maintain a diversity of forage species to provide a variety of food sources and habitats for a diversity of soil organisms
- Avoid the use of soil-applied pesticides and concentrated fertilizers that may kill or inhibit the growth of soil organisms
- Encourage animal movement across paddock for even distribution of manure nutrients
Avoid nutrient losses
- Minimize sulfur losses by using management practices that decrease the potential for leaching
- Minimize nutrient losses caused by erosion by using management practices that maintain a complete cover of forages and residues over the pasture surface
Maintain nutrient balances in the pasture
- Ensure magnesium availability to minimize the potential for grass tetany. This can be done by balancing the availability of magnesium with the availability of other soil cations, particularly potassium and calcium. Phosphorus fertilization of pastures in spring can also enhance magnesium availability.
- Guard against a build up of potassium in pastures by not overapplying manure. High potassium levels can cause reproductive problems, especially in dairy cows.
|
Back to top
Chapter 2: Nutrient Availability in Pastures
Nutrient balances and nutrient availability determine the fate of nutrients in pastures. In the simplest of grazing systems, forage crops take up nutrients from the soil; haying and animal grazing removes forage crops and their associated nutrients; and animal manure deposition returns nutrients to the soil. Continuous nutrient removals deplete soil fertility unless fertilizers, whether organic or synthetically produced, are added to replenish nutrients. Nutrients may be added to pastures by providing animals with feed supplements produced off-farm. In addition, practices that erode topsoil and deplete soil organic matter decrease the ability of soils to hold or retain nutrients.
Nutrient-depleted soils produce low-yielding forages and unthrifty animals. Excess soil nutrients can be dangerous to animal health and increase the potential for contamination of wells, springs, rivers, and streams. |
Chemical and biological interactions determine the availability of nutrients for plant use. Both native soil characteristics and land management practices affect these interactions. Phosphorus can be held chemically by iron or aluminum bonds while potassium can be held within soil minerals. All crop nutrients can be components of plant residues or soil organic matter. The type of organic matter available and the activity of soil organisms determine the rate and amount of nutrients mineralized from these materials. Nutrient availability and balance in forage plants affects the health of grazing animals. Depleted soils produce unhealthy, low-yielding forages and unthrifty animals; excess soil nutrients can be dangerous to animal health and increase the potential for contamination of wells, springs, rivers, and streams.
Soil Parent Material
Chemical, physical, geological, and biological processes affect nutrient content and availability in soils. As discussed in the previous chapter, soils derived from basalt and shale provide phosphorus to soils, granite contains high concentrations of potassium, and limestone is a source of calcium and magnesium. Some clay soils and soils with high percentages of organic matter contain a native store of nutrients in addition to having the capacity to hold nutrients added as manure, crop residues, or fertilizers. Soils formed under temperate prairies or in flood plains are fertile due to a long history of organic matter deposition and nutrient accumulation. Sandy soils and weathered, reddish clay soils contain few plant nutrients and have a limited ability to hold added nutrients. Soils formed under dry, desert conditions often are saline since water evaporating off the soil surface draws water in the soil profile upward. This water carries nutrients and salts, which are deposited on the soil surface when water evaporates. Tropical soils generally have low fertility since they were formed under conditions of high temperatures, high biological activity, and high rainfall that caused rapid organic matter decomposition and nutrient leaching.
Back to top
Soil Chemistry
Many clay minerals are able to hold onto water and nutrients and make them available for plant growth. The pH, or level of acidity or alkalinity of the soil solution, strongly influences the strength and type of bonds formed between soil minerals and plant nutrients. Soil pH also affects activities of soil organisms involved in the decomposition of organic matter and the dissolution of plant nutrients from soil minerals. Many clay soil particles are able to bind large amounts of nutrients because of their chemical composition and because they are very small and have a large surface area for forming bonds. Unfortunately, this small size also makes clay particles prone to compaction, which can reduce nutrient and water availability. Sandy soils are porous and allow water to enter the soil rapidly. But these soils are unable to hold water or nutrients against leaching. Organic matter has a high capacity to hold both nutrients and water. Soil aggregates, formed by plant roots and soil organisms, consist of mineral and organic soil components bound together in soft clumps. Aggregates enhance soil porosity, facilitate root growth, allow for better infiltration and movement of water and nutrients through soil, and help soils resist compaction.
Back to top
Prior Management Practices
In pastures, continual removal of nutrients through harvests or heavy grazing without return or addition of nutrients depletes the soil. Land management practices that encourage soil erosion—such as heavy grazing pressure, plowing up and down a slope, or leaving field bare of vegetation during times of heavy rains or strong winds—also deplete soil fertility. Some pasture management practices involve the use of fire to stimulate growth of native forages.(32) Burning readily mineralizes phosphorus, potassium, and other nutrients in surface crop residues. It also volatilizes carbon and nitrogen from residues and releases these nutrients into the atmosphere, thus minimizes the ability of organic matter to build up in the soil. Loss of residues also exposes soil to raindrop impact and erosion. Hot, uncontrolled fires increase the potential for erosion by degrading natural biological crusts formed by lichen, algae and other soil organisms and by promoting the formation of physical crusts formed from melted soil minerals.(33, 34) The continual, high application of manure, whey, sludge, or other organic waste products to soils can cause nutrients to build up to excessive levels. Pasture management practices that influence soil compaction, soil saturation, the activity of soil organisms, and soil pH affect both soil nutrient content and availability.
Back to top
Soil Compaction
Animal movement compacts soil pores, especially when soils are wet or saturated. Continuously trampling and foraging, especially in congregation areas and laneways, also depletes plant growth and produces bare spots.
Soil compaction increases as soil moisture, animal weight, animal numbers, and the length of stay in the paddock increase.
Resistance to compaction increases as forage establishment and the percentage of plants with fibrous roots increase. |
Soil compaction reduces nutrient availability for plant uptake by restricting nutrient transport to plant roots and root growth through the soil profile. Treading and compaction can substantially reduce forage yields. One study showed that the equivalent of 12 sheep treading on mixed ryegrass, white clover, and red clover pasture reduced yields by 25% on dry soil, 30% on moist soil, and 40% on wet soil compared to no treading. On wet soils, root growth was reduced 23%.(35)
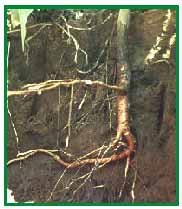
Compacted soils do not allow for normal root growth. This root grew horizontally when it encountered a compacted layer.
Photo by: Scott Bauer
2004 USDA Image Gallery |
Compaction also decreases the rate of organic matter decomposition by limiting the access soil organisms have to air, water, or nutrients. In addition, compacted soils limit water infiltration and increase the potential for water runoff and soil erosion. In Arkansas, observations of overgrazed pastures showed that manure piles on or near bare, compacted laneways were more readily washed away by runoff than were manure piles in more vegetated areas of the pasture.(24)
The potential for animals to cause soil compaction increases with soil moisture, the weight of the animal being grazed, the number of animals in the paddock, and the amount of time animals stay in the paddock. The potential for a paddock to resist compaction depends on the duration of forage establishment and the type of forage root system. Established forages with a strong and prolific root growth in the top 6 to 10 inches of the soil profile are able to withstand treading by grazing animals. Grasses with extensive fibrous root systems, such as bermuda grass, are able to withstand trampling better than grasses like orchardgrass that have non-branching roots or legumes like white clover that have taproots.(36) Bunch grasses expose more soil to raindrop impact than closely seeded non-bunch grasses or spreading, herbaceous plants. However, these grasses enhance water infiltration by creating deep soil pores with their roots.(3) Combining bunch grasses with other plant varieties can increase water infiltration while decreasing the potential for soil compaction and water runoff.
The risk of soil compaction can also be reduced by not grazing animals on paddocks that are wet or have poorly-drained soils. Instead, during wet conditions, graze animals on paddocks that have drier soils and are not adjacent to streams, rivers, seeps, or drainage ways. Soils that are poorly drained should be used only in the summer when the climate and the soil are relatively dry.
Compacted soils can recover from the impacts of compaction, but recovery is slow. Periods of wet weather alternating with periods of dry weather can reduce compaction in some clay soils. Freezing and thawing decreases compaction in soils subjected to cold weather. Taproots are effective in breaking down compacted layers deep in the soil profile while shallow, fibrous roots break up compacted layers near the soil surface.(37) Active populations of soil organisms also reduce soil compaction by forming soil aggregates and burrowing into the soil.
Back to top
Organic Matter
Nutrient release from organic matter decomposition
Manure and plant residues must be decomposed by soil organisms before nutrients in these materials are available for plant uptake. Soil organisms involved in nutrient decomposition require a balance of nutrients to break down organic matter efficiently. Manure and wasted forages are succulent materials that have high nitrogen content and a good balance of nutrients for rapid decomposition.
Time required for organic matter decomposition is affected by:
- the carbon to nitrogen ratio of organic matter
- temperature
- moisture
- pH
- diversity of soil organisms
|
Dried grasses, such as forages that died back over winter or during a drought, or manure mixed with wood bedding, have lower nitrogen contents and require more time for decomposition. In addition, soil organisms may need to extract nitrogen and other nutrients from the soil to balance their diet and obtain nutrients not available in the organic matter they are decomposing. Composting these materials increases the availability of nutrients and decreases the potential for nutrient immobilization when materials are added to the soil. Temperature, moisture, pH, and diversity of soil organisms affect how rapidly organic matter is decomposed in the soil.
Nutrient release from organic matter is slow in the spring when soils are cold and soil organisms are relatively inactive. Many farmers apply phosphorus as a starter fertilizer in the spring to stimulate seedling growth. Even though soil tests may indicate there is sufficient phosphorus in the soil, it may not be readily available from organic matter during cool springs.
Table 10: Effects of 11 Years of Manure Additions on Soil Properties |
|
Manure application rate (tons/acre/year) |
None |
10 tons |
20 tons |
30 tons |
Organic Matter (%) |
4.3 |
4.8 |
5.2 |
5.5 |
CEC (me/100g) |
15.8 |
17.0 |
17.8 |
18.9 |
pH |
6.0 |
6.2 |
6.3 |
6.4 |
Phosphorus (ppm) |
6.0 |
7.0 |
14.0 |
17.0 |
Potassium (ppm) |
121.0 |
159.0 |
191.0 |
232.0 |
Total pore space (%) |
44.0 |
45.0 |
47.0 |
50.0 |
From: Magdoff and van Es, 2000 (1) |
Nutrient holding capacity of organic matter
Besides being a source of nutrients, soil organic matter is critical for holding nutrients against leaching or nutrient runoff. Stabilized organic matter or humus chemically holds positively-charged plant nutrients (cations). The ability of soil particles to hold these plant nutrients is called cation exchance capacity or CEC. Continual application of organic materials to soils increases soil humus (38) and enhances nutrient availability, nutrient holding capacity, and soil pore space.
Soil aggregates
Soil humus is most effective in holding water and nutrients when it is associated with mineral soil particles in the form of soil aggregates. Soil aggregates are small, soft, water-stable clumps of soil held together by fine plant root hairs, fungal threads, humus, or microbial gels. Aggregates are also formed through the activities of earthworms. Research has shown that several species of North American earthworms annually consume 4-10% of the soil and 10% of the total organic matter in the top 7 inches of soil.(39) This simultaneous consumption of organic and mineral matter by earthworms results in casts composed of associations of these two materials. Earthworms, as well as dung beetles, incorporate organic matter into the soil as they burrow.
Besides enhancing the nutrient and water holding capacity, well-aggregated soils facilitate water infiltration, guard against runoff and erosion, protect against drought conditions, and are better able to withstand compaction than less aggregated soils. Since aggregated soils are more granular and less compacted, plant roots grow more readily through them and air, water, and dissolved plant nutrients are better able to flow through them. These factors increase plant access to soil nutrients.
To enhance aggregation within pasture soils, maintain an optimum amount of forages and residues across paddocks, avoid the formation of bare areas, and minimize soil disturbance. Grazing can degrade soil aggregates by encouraging mineralization of the organic glues that hold aggregates together. In areas with a good cover of plant residues, animal movement across pastures can enhance aggregate formation by incorporating standing dead plant materials into the soil.(40)
Back to top
Soil pH
Soil mineralogy, long-term climate conditions, and land-management practices affect soil pH. The acidity or alkalinity of soils affects the nutrient availability, nitrogen fixation by legumes, organic matter decomposition by soil organisms and plant root function. Most plant nutrients are most available for uptake at soil pH in the range of 5.5 to 6.5. Legume persistence in pastures is enhanced by soil pH in the range of 6.5-7.0. In low-pH or acid soils, aluminum is toxic to root growth, aluminum and iron bind phosphorus, and calcium is in a form with low solubility. In high-pH or alkaline soils, calcium carbonate binds phosphorus while iron, manganese, and boron become insoluble.
Application of some synthetic nitrogen fertilizers acidifies soils. Soil microorganisms involved in nitrification rapidly transform urea or ammonium into nitrate nitrogen. This nitrification process releases hydrogen ions into the soil solution, causing acidification, which decreases nutrient availability, thus slowing the growth of plants and soil organisms. Nitrification also occurs in urine patches when soil microorganisms transform urea into nitrate.
Lime soils with calcium carbonate if the soil is low in calcium. Use dolomite limestone if grass tetany or magnesium deficiency is an animal health problem. |
Another fertilizer that acidifies the soil is superphosphate. Superphosphate forms a highly acid (pH 1.5) solution when mixed with water. The impact of this acidification is temporary and only near where the fertilizer was applied, but, in this limited area, the highly acid solution can kill rhizobia and other soil microorganisms.(9)
The type and diversity of forage species in pastures can alter soil pH. Rangeland plants such as saltbush maintain a neutral soil pH, while grasses and non-legume broadleaf plants tend to increase pH and legumes tend to decrease it. The impact of plant species on pH depends on the type and amounts of nutrients they absorb. Rangeland plants absorb equal amounts of cation (calcium, potassium, magnesium) and anion (nitrate) nutrients from the soil. Grasses and non-legume broadleaf plants absorb more anions than cations since they use nitrate as their primary source of nitrogen. Legumes that actively fix nitrogen use very little nitrate. Consequently, they reduce the pH of the soil since they take up more cations than anions.(9) A combination of legumes and non-legumes will tend to stabilize soil pH.
Pasture soils should be tested regularly to determine soil nutrients, soil organic matter and pH. Based on test results and forage nutrient requirements, management practices can adjust soil pH. Lime and organic matter increase soil pH and decrease soil acidity. Soil organic matter absorbs positive charges, including hydrogen ions that cause soil acidity.(41) Lime increases soil pH by displacing acid-forming hydrogen and aluminum bound to the edges of soil particles and replacing them with calcium or magnesium. Limestone that is finely ground is most effective in altering soil pH since it has more surface area to bind to soil particles. All commercial limestone has label requirements that specify its capacity to neutralize soil pH and its reactivity based on the coarseness or fineness of grind.
Lime refers to two types of materials, calcium carbonate and dolomite. Dolomite is a combination of calcium and magnesium carbonate. Calcium carbonate is recommended for soils low in calcium; where grass tetany or magnesium deficiency is an animal health problem, dolomite limestone should be used. In sandy soils or soils with low to moderate levels of potassium, the calcium or magnesium in lime can displace potassium from the edges of soil particles, reducing its availability. Therefore, these soils should receive both lime and potassium inputs to prevent nutrient imbalances.
Back to top
Timing of Nutrient Additions
The timing of nutrient additions to fields or pastures determines how effectively plants take up and use nutrients while they are growing and setting seed. Different nutrients are important during different stages of plant development. Nitrogen applied to grasses before they begin flowering stimulates tillering, while nitrogen applied during or after flowering stimulates stem and leaf growth.(9) However, fall nitrogen applications for cool season grasses are more effective and economical than spring applications. In most years, nutrient releases through mineralization are sufficient to stimulate forage growth in the spring. Applications of nitrogen in the late summer and fall allow cool season grasses to grow and accumulate nutrients until a killing frost. This provides stockpiled growth for winter grazing.(42)
Both potassium and phosphorus are important for increasing the nutrient quality of forages, extending stand life, and enhancing the persistence of desirable species in the forage stand.(42) Phosphorus is critical for early root growth, for seed production, and for effective nitrogen fixation by legume nodules. Potassium is important during the mid-to-late growing season. It increases the ability of plants to survive winter conditions, by stimulating root growth and reducing water loss through stomata or leaf pores.(43) It also is important for legume vigor and enhancing plant disease resistance.(42)
Nutrient uptake by plants corresponds to their growth cycle. Warm season forages exhibit maximal growth during the summer while cool season forages exhibit maximum growth during the spring and early fall.(32) Pastures containing a diverse combination of forages will use nutrients more evenly across the growing season while less diverse pastures will show spikes in nutrient uptake requirements.
Nitrogen fertilization depresses nitrogen fixation by legumes since they require less energy to take up nitrogen from the soil than they need to fix nitrogen. |
Legumes provide nitrogen to the pasture system through their relationship with the nitrogen-fixing bacteria, rhizobia. If nitrogen levels in the soil are low, newly planted legumes require nitrogen fertilization until rhizobia have developed nodules and are able to fix nitrogen. Once they start fixing nitrogen, nitrogen fertilization depresses nitrogen fixation by legumes since they require less energy to take up nitrogen from the soil than they need to fix nitrogen. Legumes can fix up to 200 pounds of nitrogen per year, most of which becomes available to forage grasses in the following years. Phosphorus is essential for effective nodule formation and nitrogen fixation. In acid soils, liming may make phosphorus already in the soil more available, thereby decreasing the need for fertilization.
As discussed above, the type of organic material added to the soil, as well as temperature, moisture, pH, and diversity of soil organisms, determines how rapidly soil organisms decompose and release nutrients from organic matter. Synthetic fertilizers are soluble and immediately available for plant uptake. Therefore, these fertilizers should be applied as close as possible to when plants can use nutrients for growth. A lag time of 2-21 days may pass after fertilizers are applied before increased forage production is observed.
A lag time of 2-21 days may pass after fertilizers are applied before increased forage production is observed. |
Organic material releases nutrients over a period of several years. On average, only 25-35% of the nitrogen in manure is mineralized and available for plant use during the year of application. Another 12% is available in the following year, 5% in the second year following application, and 2% in the third year.(44) Manure deposited in pastures causes an increase in forage growth approximately 2-3 months after deposition with positive effects on growth extending for up to two years.(11) Alfalfa can supply approximately 120 pounds of nitrogen to crops and forages in the year after it is grown, 80 pounds of nitrogen during the following year, and 10-20 pounds in the third year.(44) Due to this gradual release of nutrients from organic materials, continual additions of manure or legumes will compound the availability of nutrients over time. Accounting for nutrients available from previous years is critical for developing appropriate application rates for manure and fertilizers during each growing season. Not accounting for these nutrients can result in unnecessary fertilizer expenses and risks of nutrient losses to the environment.
Nutrients from both organic and synthetic fertilizers can be lost through leaching, runoff, or erosion. The potential for nutrient losses is greatest if these materials are applied in the fall or winter, when plants are not actively growing, or during times when soils are frozen, snow-covered, or saturated. During times of high rainfall, nitrate may leach through the soil since it does not bind to soil particles. Rainfall also facilitates the transport of phosphorus in runoff water or through artificial drainage tiles to water bodies. Rainfall or snowmelt water flowing over bare soil causes soil erosion and the transport of nutrients attached to soil particles.
Table 11: Pasture Management Practices for Enhancing Nutrient Availability
Ensure plant cover and diversity across pastures
- Use management practices that maintain a complete cover of forages and residues across pastures
- Combine bunchgrass species with a diversity of forage species, including plants with prostrate growth habit to provide both good water infiltration and protection against erosion and soil compaction
Grazing management practices during wet weather
- Use well-drained pastures or a "sacrificial pasture" that is far from waterways or water bodies
- Avoid driving machinery on pastures that are wet or saturated
- Avoid spreading manure or applying fertilizers to soil that is saturated, snow-covered, or frozen
Ensure effective use of nutrient inputs
- Use management practices that encourage the even distribution of manure and urine across paddocks
- Rely on soil tests and other nutrient management practices when applying fertilizers and manure to pastures
- Account for nutrients available from manure and legume applications during prior years when developing fertilizer or manure application rates for the current year
- Sample the nutrient content of added manure to determine appropriate rates of application
- Choose the appropriate type of limestone to apply for pH adjustment based on calcium and magnesium needs and balances in pastures
- Either avoid the use of fertilizers that decrease soil pH or use lime to neutralize soils acidified by these fertilizers
- Apply nitrogen fertilizer in the fall to enhance the amount of forages stockpiled for winter grazing
- Apply sufficient phosphorus and potassium while limiting additions of nitrogen in order to favor growth of legumes in your pastures
|
Back to top
Chapter 3: Nutrient Distribution and Movement in Pastures
Farmers and ranchers graze animals using a variety of management methods. In this document, extensive grazing refers to the practice of grazing animals continuously or for extended periods of time on a large land area. Rotational grazing is a management intensive system that concentrates animals within a relatively small area (a paddock) for a short period of time, often less than a day for dairy animals. The animals are then moved to another paddock, while the first paddock is allowed to recover and regrow. Animals are moved according to a flexible schedule based on the herd size, the amount of land available, quality of forages in the paddock, and forage consumption. Grazing managers determine when and how long to graze animals in specific paddocks based on climatic conditions, soil characteristics, land topography, and the distance the paddock is from streams or rivers.
Pasture size, shape, and topography, stocking rate, grazing duration, and time of day all affect how animals graze, lounge, drink water, and use feed or mineral supplements. Also, different grazing animal species vary in their use of nutrients and herding behavior. These factors, along with soil characteristics, climate, and forage and soil management practices, affect nutrient cycling in pastures, animal growth and productivity, and potential of manure nutrients to contaminate ground or surface water.
Pasture Nutrient Inputs and Outputs
Nutrient balances
Figure 7. Pasture Nutrient Inputs and Outputs
|
From Klausner, 1995 (44) |
Maintaining a balance between nutrients being removed from pastures and nutrients being returned to pastures is critical to ensure healthy and productive forage growth as well as control of nutrient runoff and water body contamination. Nutrient balances in pastures are determined by subtracting nutrient removals in the form of hay harvested, feed consumed, and animals sold from nutrient inputs including feed, fertilizer, and manure.
Grazing animals that receive no mineral or feed supplements will recycle between 75-85% of forage nutrients consumed. If no fertilizer or outside manure inputs are applied, continual grazing will cause a gradual depletion of plant nutrients. Animals provided feed or mineral supplements also deposit 75-85% of the nutrients from these inputs as urine or feces.(42) These nutrients represent an input into the pasture system. Nutrient inputs from non-forage feeds can be substantial for dairy and other animal operations that use a high concentration of grain and protein supplements, importing into the pasture approximately 148 pounds N, 32 pounds P, and 23 pounds K per cow per year.(42) Winter feeds also form a substantial input into the pasture nutrient budget when animals are fed hay while being kept on pasture.
Manure deposition and distribution
A cow typically has 10 defecations per day, with each manure pile covering an area of approximately 1 square foot.(47) They will also urinate between 8 to 12 times per day.(48) Each urination spot produces a nitrogen application equivalent to 500-1,000 pounds/acre while each defecation represents a nitrogen application rate of 200-700 pounds/acre.(42) An even distribution of nutrients throughout a paddock is required for productive plant and animal growth. Unfortunately, grazing animals do not naturally deposit urine and feces evenly across the paddocks where they graze. In one rotational grazing study, urine spots occupied 16.7% of the pasture while manure spots occupied 18.8%, following 504 grazing days per acre.(49) Intensity of grazing rotations affects the distribution of manure coverage in paddocks. Under continuous, extensive grazing practices, 27 years would be needed to obtain one manure pile on every square yard within a paddock; if a two-day rotation were used instead only two years would be needed.(42)
Table 12: Nutrient Consumption and Excretion by Grazing Animals |
|
Dairy cows |
Beef / Sheep |
Feed consumption / day |
18-33 lbs. |
|
Nutrients used for growth and reproduction |
17% N
26% P |
15-25% N
20% P, 15% K |
Nutrients removed from pasture in form of milk and meat |
N-84 lbs. / cow
P-15 lbs. / cow
K-23 lbs. / cow |
N-10 lbs. / cow-calf
P-3 lbs. / cow-calf
K-1 lbs. / cow-calf |
Nutrients/ton manure |
6-17 lbs. N
3-12 lbs. P2O5
2-15 lbs. K2O |
|
Nitrogen in feces |
2.0-3.6% |
3.4-3.6% |
Nitrogen excreted as feces |
55 lbs. / year |
|
Nitrogen in urine |
0.42-2.16% |
0.30-1.37% |
Nitrogen excreted as urine |
165 lbs. / year |
|
From: Stout et al (45), Detling (46), Russelle (17), Wells and Dougherty (47), Haynes and Williams (41), Klausner (44), Lory and Roberts (42) |
Nutrient concentration within pastures is due to the tendency of grazing animals to congregate. They tend to leave manure piles or urine spots around food and water sources, on side hills, in depressions, along fence lines, and under shade. Sheep have a greater tendency than cattle to congregate and deposit manure in these areas.(50) Prevailing wind direction and exposure to sunlight can also affect animal movement, congregation, and manure deposition.(51) Laneways between pastures and leading to watering areas are another area of animal congregation and manure deposition. When animals have to walk over 400 feet from the pasture to water, they deposit between 13%-22% of their manure on laneways.(47, 52)
A study conducted in Iowa showed a buildup of nutrients extending 30 to 60 feet into the pasture around water, shade, mineral supplements, and other areas where cattle congregated.(53) Nutrients are concentrated in these congregation areas because animals transport nutrients from areas where they graze. Consequently, they also deplete nutrients from the grazing areas. Grazing practices that encourage foraging and manure distribution across paddocks and discourage congregation in limited areas will improve nutrient balances within pastures.
The time of day that animals congregate in different areas determines the amount and type of nutrient that accumulates in each area. Animals tend to deposit feces in areas where they rest at night or ruminate during the day while they urinate more in the areas where they graze during the day.(47) Nitrogen is present in both feces and urine while phosphorus is primarily deposited as feces, and potassium is predominately found in urine. While most urine is deposited during the day, urine that is deposited at night has a higher nutrient content than urine deposited while grazing.(41) Due to these factors, phosphorus will accumulate in resting areas (13) while nitrogen and potassium will accumulate in both resting and grazing areas.
Minimizing animal congregation
By working with the normal foraging and herding behavior of grazing animals, distribution of animals across paddocks can be encouraged. In larger paddocks, animals tend to graze and lounge as a herd, while they distribute themselves more evenly across smaller paddocks.(41) In larger paddocks, animals visit water, minerals, shade and fly control devices as a herd while animals concentrated within small paddocks tend to visit these areas one-by-one. Locating nutrients, shade, and pest control devices farther apart in the paddock further discourages concentration of animals and manure. If a particular area of a paddock is deficient in nutrients, placement of supplemental feeds in that area can be used to encourage congregation and manure deposition there.
Figure 8. 3-Paddock System
|
|
Figure 9. 12-Paddock System
|
|
Figure 10. 24-Paddock System
|
Subdividing depressions, side hills, and shady areas among several paddocks can enhance nutrient distribution across the landscape. Research conducted in Missouri showed that manure nutrients were distributed more evenly across the landscape when a field was managed using 12 or 24 paddocks rather than only 3 paddocks.(54) Animals in the smaller paddocks concentrated around favored areas for less time than did animals in larger paddocks. Since animals tend to graze along the perimeter of fence lines, they distribute nutrients most evenly across paddocks that are small, square, and have water available.(55) An efficiently designed paddock allows animals to graze and drink with a minimum amount of time, effort, and trampling of the pasture sod.
Back to top
Manure Nutrient Availabilty
Feces contain nitrogen predominately in the organic form, while 60-70% of cow urine nitrogen and 70-80% of sheep urine nitrogen is in the form of urea. Urea and potassium in urine are soluble and therefore immediately available for plant uptake. Phosphorus in feces is predominantly in the organic form and must undergo decomposition before it is available to plants. Soil organisms will relatively quickly decompose moist, nitrogen-rich manure piles derived from succulent grasses. They will have difficulty breaking down manure that derived from hay or older forages that is stiff, dry and crusty. When a hard crust forms on manure piles during dry weather, both physical breakdown and biological decomposition are inhibited.(41) By treading on manure piles as they move around a pasture, animals physically break down manure piles into smaller pieces that are more easily consumed by soil organisms.
Because nutrients are released slowly from manure, forage plants in the vicinity of manure piles will grow slowly for about two months following manure deposition.(41, 42) However, as decomposition of manure piles by soil organisms makes nutrients available for plant use, greater pasture regrowth and forage production occurs in the vicinity of manure and urine compared to other pasture areas.(49, 54, 56) Increases in nitrogen availability in areas near manure piles can favor the growth of grasses compared to legumes (9), an impact that can last for up to two years.(41)
Animals naturally avoid grazing near dung sites, but will feed closer to manure piles (41) and use forages more efficiently as grazing pressure intensifies. In multispecies grazing systems, sheep do not avoid cattle manure as much as cattle do.(57) While both sheep and cattle avoid sheep manure, the pellet form of sheep manure has a large surface area, and thus breaks down more rapidly than cattle manure. Consequently, forages are used more effectively when cattle are combined with either sheep or goats.
Back to top
Pasture Fertilization
Manure and fertilizers are applied to pastures to provide nutrients necessary to obtain effective plant growth and animal production. Applications should be based on regular soil testing, the ability of soil to provide and retain nutrients, plant needs, grazing intensity, and nutrient removals through haying. Prior to the current concerns over water quality, manure application recommendations were made to meet forage plant needs for nitrogen. Continued nitrogen-based applications result in a phosphorus buildup in the soil since manure usually contains about the same concentration of phosphorus and nitrogen, while plants only require one-half as much phosphorus as nitrogen. In diverse pastures that contain a combination of grasses and legumes, decreasing or eliminating manure applications can lower phosphorus imbalances while maintaining forage yields. Nitrogen-fixing capabilities of legumes reduce forage nitrogen requirements while using excess soil phosphorus.
Fertilizer and manure applications should be based on regular soil testing, the ability of soil to provide and retain nutrients, plant needs, and grazing intensity. |
On some farms, manure is applied to soil as a waste product. Instead of being applied according to crop needs, manure is primarily applied according the need to dispose of manure, the location of field in relation to the barn, and the accessibility of field during bad weather. These "waste application" practices present a high potential for nutrient buildup and movement of excess nutrients to ground or surface waters.
Figure 11. P Added in Manure/Removed by Crop
|
Applying manure to meet the nitrogen needs of corn (about 200 lbs. N/acre) adds much more phosphorus than corn needs.

|
From Sharpley, et al. (58) |
To ensure that manure is used effectively as a source of plant nutrients and poses minimal risks to the environment, it should be applied according to a nutrient management plan. Natural Resource Conservation Service and Soil and Water Conservation District personnel as well as many commercial crop consultants are trained in the development of nutrient management plans. Software programs to develop your own nutrient management plan may be available from Cooperative Extension Service educators or Agronomy and Soil Science specialists at land grant universities.
Table 13: Components of a Comprehensive Nutrient Management Plan
Components of a Comprehensive Nutrient Management Plan
- Soil tests on all fields or pastures
- Manure tests
- Load capacity and rate of application of manure spreading equipment
- Timing and method of manure and fertilizer applications
- Prior land management practices including manure applications, legumes used as green manures, fallows, or hay removal
- Assessments of runoff, erosion, and flooding potentials for each field or paddock
- Crops or forages to be produced
- Current pasture management practices including stocking rates and hay removal
Format of a nutrient management plan for each paddock or field
- Soil and manure test results
- Risk factors such as excess nutrient levels, high runoff, erosion, or flooding potential
- Recommended time, method, and rate for fertilizer and manure applications
- Recommended time for grazing, especially on pastures with moderate to high potentials for runoff, erosion, or flooding
- Management practices to minimize risk factors and maximize nutrient availability to forages
|
Back to top
Grazing Intensity
Definition
Grazing intensity refers to the impact animals have on forage growth and reproduction and on soil and water quality. It is influenced by animal foraging habits, stocking rates, the length of time animals are allowed to graze within a given paddock, and the relation these factors have on soil characteristics and climatic conditions. Continuous high-intensity grazing depletes soil nutrients, decreases the diversity of forage species, inhibits the ability of some forage plants to regrow and reproduce, and increases the potential for nutrient runoff and erosion. Conversely, short-term high-intensity grazing combined with a resting period (as in rotational grazing practices) causes an increase in the diversity of forage species, enhances the dispersal of forage seeds, and helps conserve nutrient resources within the soil-plant system.
Short-term high-intensity grazing combined with a resting period (as in rotational grazing practices) causes an increase in the diversity of forage species, enhances the dispersal of forage seeds, and helps conserve nutrient resources within the soil-plant system. |
Grazing behavior, plant growing points, and plant leaf area
Grazing habits of different animal species have different impacts on forage species composition in pastures. For example, horses graze more closely to the ground than cattle; sheep graze at soil level and can take away the base of grass plants below the area of tiller emergence (59); while cattle tend to graze taller grasses that sheep may reject. Animal grazing behavior, the location of a plant's growing point, and the amount of leaf area remaining when animals are rotated to another pasture affects the ability of plants to regrow. If grazing animals remove the growing point and substantial leaf area of grasses, new leaf growth must come from buds that have been dormant and the energy for this growth must come from stored carbohydrates rather than from photosynthesis.(60)
Early in the growing season, all grasses have their growing points at or near the ground level. Ryegrass, tall fescue, Kentucky bluegrass and many other species of cool-season grasses have growing points that remain at or below ground level throughout most of the growing season. Other species of predominantly native grasses, including smooth broomgrass, timothy, reed canarygrass, switchgrass, and gamagrass have stems that elongate below the growing point above the soil level.(60) As long as the growing point remains intact, the plant is capable of producing new leaves. Grasses with low growing points are able to recover from grazing relatively quickly because the growing point is not disturbed. If the growing point is removed, growth results from the emergence of new tillers. Under continuous, intensive grazing practices, warm-season grasses recover more slowly than cool-season grasses, especially during the spring.(61) As a result, continuous grazing practices or grazing too early in the season tends to favor the growth of non-native grasses and decrease the diversity of forages in pastures.(62)
Continuous grazing tends to favor the growth of cool-season grasses since grazing animals remove the elevated growing points of native warm-season grasses more readily than they remove the lower growing points of non-native, cool-season grasses. |
For areas with moderate rainfall, leaf area remaining after grazing is more critical for forage recovery than the location of a forage plant's growing point (J. Gerrish, personnal communication). Most forbs and legumes, such as alfalfa and red clover, have aerial growing points relatively high up on the plant, which are easily removed by grazing animals. This is not detrimental to plant growth unless a majority of the leaf area or the basal portion of the plant is removed. For optimal recovery, at least 3-4 inches of residual leaf area should remain on cool-season grasses while 4-8 inches of leaf area should remain for warm-season grasses following grazing.(61) Other factors that affect plant regrowth include treading impact on leaf and root growth, forage composition impact on the ability of plants to intercept sunlight for photosynthesis, and soil conditions.(35)
Nutrient uptake
Forage plants that are cut or regrazed frequently during the growing season take up more nutrients than forages that are not cut or grazed. Research conducted in Kansas indicated that cutting pasture forage six times during the growing season resulted in 4.3 times greater nitrogen content and 5.2 times greater phosphorus content in cut forages compared to uncut plots.(63) Cutting pastures in the spring when seed heads are forming can also increase the productivity and nutrient uptake of pasture forages.(64) Other studies (56, 65) demonstrated that increased grazing intensity resulted in younger, more succulent plants with a higher nitrogen content compared to plants growing in ungrazed areas. The higher nitrogen content was attributed to return of nitrogen to the system through urine and to the availability of nitrogen fixed by legumes. In these studies legumes remained prevalent in the more intensely grazed plots while their populations decreased in the more lightly grazed paddocks.(65)
Yield
During the first year of intensive grazing, increasing the intensity of cutting or grazing increases the amount of forage produced. Following grazing, photosynthesis is stimulated and plants take up more nutrients. This permits leaf regrowth in broadleaf plants and increased tillering in grasses. Increased leaf area then allows for greater photosynthesis. As photosynthesis and the formation of carbohydrates increases, nutrient uptake by roots and subsequent movement of nutrients from roots to leaves also increases. However, as more energy and nutrients are allocated to leaf production and increased photosynthesis, less energy and nutrients are provided for root growth.(63)
Frequently grazed plots exhibit high biomass production and nutrient uptake during the initial grazing season. But if grazing intensity is too great, forage production will decrease in the following years. |
While frequently mowed or grazed plots exhibit high biomass production and nutrient uptake during the initial grazing season, if the intensity of grazing is too high, forage production will decrease in following years (63, 66). This production decline is due to decreased plant ability to take up nutrients from the soil due to decreased root growth, as well as to a depletion of soil nutrients. Severe grazing will also affect plant diversity since grazing during flowering removes seed heads and flowers, limiting the reseeding of forage plants.(64)
A sufficient resting period allows plants to regrow and produce adequate leaf area for photosynthesis. It also allows plants and soil organisms to reduce soil compaction and increase the availability of nutrients through mineralization. |
Root growth is critical for water and nutrient uptake. Plants can also store food reserves in roots to allow for regrowth during periods of stress. Plants grazed too frequently or cut too short have difficulty producing more leaves because of limited growth and food reserve storage by roots. In one study, plants that were not cut until they reached 8 inches tall produced more growth than did plants cut every time they reached 2 inches tall. Similarly, grasses subjected to continuous intensive grazing by sheep produced less vegetation than lightly grazed pastures. In both cases, a longer resting period resulted in better plant growth, since the resting period allowed plants to regrow and produce adequate leaf area for photosynthesis.(63) Grass tiller population and pasture production markedly increased in an extensively grazed pasture that was fallowed for one year. This resting period allowed plants and soil organisms to reduce soil compaction and increase the availability of nutrients through mineralization.(67)
Cutting grasses short not only depresses plant regrowth, it also increases soil temperature. As soil temperature increases so does nutrient mineralization by soil organisms. While mineralization is necessary to release nutrients from plant and animal residues, if mineralization is too rapid, it can cause a loss of organic matter.(65) Grazing or cutting pastures too short can also expose bare soil to the impact of rainfall, increasing the potential for soil compaction and the loss of topsoil and nutrients through erosion. Nutrient cycling and effective nutrient use by plants depend on pasture management practices that minimize soil compaction, conserve organic matter, and do not hinder plant regrowth following grazing.
Nutrient cycling and effective nutrient use by plants depend on pasture management practices that minimize soil compaction, conserve organic matter, and do not hinder plant regrowth following grazing. |
The nutrient content of forage plants affects animal feeding habits, the amount of nutrition animals obtain, and the type of manure they produce. Succulent, nutritionally balanced pastures provide good animal productivity and cause animals to deposit moist manure piles.(36) Animals feeding on dry, older, or overgrazed forages will obtain limited nutrient value. Manure piles produced from these forages will be stiff because of their high fiber content. Dry, stiff manure piles are difficult for soil organisms to decompose since there is little air within the pile.(68) Conversely, animals often deposit very liquid manure as they begin feeding on pastures in the spring after a winter of eating hay. The high moisture content of the pasture forages results in a very wet manure pile that disperses across the soil. Soil organisms are able to decompose manure that has relatively high nitrogen and moisture content more readily than manure that is drier and more carbon-rich.
Back to top
Diversity and Density of Pasture Plants
Figure 12. Root Growth
|
Root growth of alfalfa under irrigated (left) and dry (right) conditions (Weaver, 71) |
Diverse forage mixtures of both broad-leaved plants and grasses use solar energy efficiently. The shape and orientation of plant leaves affects how and when the plant can best conduct photosynthesis. Tall plants and upright grasses capture light at the extreme angles of sunrise and sunset while horizontal leaves of broad-leaf plants use sunlight better at midday. A combination of tall, sun-loving plants with shorter shade-tolerant plants allows for the capture of both direct and filtered sunlight. A combination of warm- and cool-season grasses allows for effective photosynthesis throughout the growing season. Warm-season grasses like big bluestem are better able to grow and use solar energy at temperatures between 90 and 100 degrees F, while cool season grasses like tall fescue grow best between 75 and 90 degrees F.(32)
Persistence of pasture legumes
Maintaining legumes as part of the forage mix is necessary if nitrogen fixation is to provide most of the nitrogen input into the pasture system. Legumes with a deep taproot and a woody crown, such as alfalfa, red clover, and birdsfoot trefoil, are able to persist in a well-drained pasture because they are able to obtain water and nutrients from deep below the soil surface. They also tolerate drought and cold, and are able to regrow unless their growing points are elevated and exposed to defoliation. Rotational grazing has been shown to increase the proportion of red clover and alfalfa in mixed pastures.(69)
White clover has rhizomes rather than a taproot. This growth habit allows it to colonize bare soils (64) by forming additional plants through the growth of stolons. White clover is competitive with grass at low production densities while legumes with taproots are more competitive at high production densities.(70)
Timing of grazing affects species composition and diversity in pastures. |
The diversity of forage species also affects the persistence of legumes within a pasture. When six to eight forage species were planted together in a Missouri pasture, pasture plant diversity remained after three years of grazing. Pastures with a diversity of forage species also maintained a higher percentage of forage cover during this time than did pastures planted to monocultures or simple mixtures of forages.(72) Productivity within pastures is more stable when forages represent a diversity of function and structure, such as height, root growth habit, life cycle, and habitat requirements.(4)
Nitrogen transfer between grasses and legumes is greatest when there is a close population balance between these species and they are growing close together. |
Nitrogen fixation is directly related to the ability of legumes to accumulate energy through photosynthesis. Thus, leaf removal decreases nitrogen fixation and leaf regrowth increases the potential for nitrogen fixation. Legumes not only fix nitrogen for their own needs, but are also able to supply nitrogen to non-nitrogen-fixing forage crops. They primarily supply nitrogen to forage plants following decomposition. Pastures dominated by clover produce around 200 pounds nitrogen per acre per year through nitrogen fixation.
Legumes can also provide nitrogen to companion grass species during the growing season. In New Zealand, perennial ryegrass obtained 6-12% of its nitrogen from associated white clover. Alfalfa and birdsfoot trefoil provided up to 75% of the nitrogen used by reed canarygrass in Minnesota. This nitrogen transfer occurs when roots die, nodules detach, or neighboring grasses and legumes become interconnected by their roots or through mycorrhizae. Nitrogen transfer between grasses and legumes is greatest when there is a close population balance between these species and they are growing close together.(15) In the first year of legume establishment, nitrogen transfer is relatively low and is derived predominantly from nodule decomposition; it increases in the second year as direct transfer mechanisms through mycorrhizae become established.(14)
Nutrient use efficiency
A diverse plant community uses soil nutrients more effectively than a monoculture or simple plant mixtures. Native grasses have a lower requirement for nitrogen and subsequently a lower concentration of nitrogen in their leaf tissue compared to non-native cool season grasses. As a result, these grasses thrive under low nutrient conditions but they provide lower-quality feed and recycle nutrients more slowly back to the soil system. The low nitrogen content of the plant litter results in slow decomposition, immobilization of nitrogen by organisms involved in decomposition, and a decrease in the nitrogen available for plant uptake.
Just as a diverse plant canopy covers the entire soil surface, a diversity of root systems occupies the entire soil profile. |
Broadleaf plants require higher nitrogen inputs for productive growth and have higher nitrogen content in their plant tissues than grasses.(73) Due to their high nitrogen content, decomposition of residues from these plants stimulates the mineralization or release of nutrients into the soil solution. Cool-season and warm-season forages grow and take up nutrients at different times of the year. A combination of cool- and warm-season forages ensures a relatively even uptake of nutrients throughout the growing season.
Just as a diverse plant canopy covers the entire soil surface, a diversity of root systems occupies the entire soil profile from the soil surface to as far as 15 feet deep. Grasses generally have fine, bushy roots. Legumes such as alfalfa or red clover have taproots. Some plants have longer or deeper root systems while other plants have a root system that grows primarily in the surface soil. Pastures that contain plants with a diversity of root systems will be better able to harvest and use nutrients from the soil than a less-diverse community. Plants with more shallow roots are effective in recycling nutrients released through the decomposition of thatch and manure on the soil surface, while deep-rooted plants are able to scavenge nutrients that have been leached down through the soil profile.
Table 14: Enhance Nutrient Balances Within the Farm and Across Paddocks
Balance nutrient inputs and outputs
- Replenish nutrients removed by grazing animals
- Recognize that feed supplements, particularly for dairy cows, represent significant nutrient inputs onto farms
- Replace nutrients based on a comprehensive nutrient management plan that takes into account prior manure additions, nitrogen contributions from legumes, and soil tests
- Apply manure based on the phosphorus needs of forages in order to avoid phosphorus build up on pastures; rely on legumes to supply much of the nitrogen needed for forage growth
Promote even distribution of manure nutrients across paddocks
- Subdivide pastures to distribute congregation areas among several paddocks
- Keep paddock dimensions as close to square as possible
- Provide animals with water in every paddock; avoid use of laneways to access water
- Locate nutrient supplements, shade, water, and pest control devices far apart from one another
Enhance nutrient availability
- Enhance growth of soil organisms involved in the decomposition of manure by maintaining good soil quality and minimizing use of soil-applied insecticides and high-salt-containing fertilizers
- A combination of cattle and sheep enhances the amount of land available for grazing since sheep graze closer to cattle manure than cattle do and feed on coarser vegetation than cattle will use
Encourage a diversity of forage species within paddocks
- Maintain a diversity of forages representing a variety of leaf and root growth habits, life cycles, and habitat preferences
- Rotate pastures while at least 4 inches of the leaf area remains. This allows plants to regrow rapidly and roots to recover
- Maintain a high percentage of legumes in the forage mix by not overgrazing and by minimizing nitrogen fertilizer additions
- Provide paddocks with sufficient rest time to allow forages to regrow
|
Back to top
Chapter 4: The Soil Food Web and Pasture Soil Quality
Diversity of the Soil Food Web
Soil organisms play a critical role in nutrient cycling. Not only are they responsible for decomposing organic matter, formation of soil aggregates, solubilizing mineral nutrients, and adjusting soil pH; they are also responsible for nitrogen fixation, nitrification, phosphorus uptake through mychorrizae, degradation of soil minerals, and formation of plant hormones. A healthy soil contains millions of organisms, ranging from visible insects and earthworms to microscopic bacteria and fungi. An acre of living soil may contain 900 pounds of earthworms, 2,400 pounds of fungi, 1,500 pounds of bacteria, 133 pounds of protozoa, and 890 pounds of arthropods and algae, as well as small mammals. The term soil food web refers to the network of dynamic interactions among these organisms as they decompose organic materials and transform nutrients.
Soil food web refers to the network of dynamic interactions among these organisms as they decompose organic materials and transform nutrients. |
While some of the organisms in this diverse community are plant pests, many more serve as antagonists of plant pests and diseases. Other soil organisms, particularly bacteria, are able to use toxic chemicals, such as pesticides, as a source of food. As they consume these toxic chemicals, they break them down into substances, such as carbon dioxide, water, and atmospheric nitrogen, that are either not toxic or are less-toxic to plants, animals, and humans.
Figure 13. Foodweb of Grassland Soil

|
From Killham, 1994 (74) |
Back to top
Organic Matter Decomposition
Soil organisms
Many soil organisms are involved in the decomposition of organic matter. Larger soil organisms, including small mammals, insects, and earthworms, are primary decomposers, involved in the initial decomposition and cycling of nutrients. Primary decomposers make greater use of carbon than of nitrogen, in their growth and respiration processes. As a result, the feces and casts deposited by them have a lower carbon content and a lower ratio of carbon to nitrogen than the original organic matter. By transforming organic matter into a simpler chemical form as well as physically breaking it down into smaller pieces, primary decomposers make these materials more available to microorganisms or secondary decomposers for further breakdown. In addition, the dead bodies of earthworms and insects are high in nitrogen and easily decomposed by soil microorganisms.(75) Fungi and bacteria are predominant secondary decomposers, however algae, protozoa, amoebae, actinomycetes, and nematodes also play important roles in transforming soil nutrients.
The chemistry of organic materials and environmental conditions determines how rapidly organic matter is broken down, which soil organisms are involved in the decomposition process, and whether organic matter decomposition will cause an initial decrease or increase in available nutrients. The soil environment determines which soil organisms are dominant and which soil organisms are less active. Some bacterial species thrive under flooded, anaerobic conditions but most soil organisms require access to oxygen. Earthworms and some soil insects require soil that is aggregated and relatively uncompacted so they can burrow through it. Environments with limited nitrogen availability are dominated by organisms that are able to fix nitrogen from the atmosphere, such as algae, lichens, and rhizobia associated with legumes. Many soil organisms are killed by non-specific insecticides as well as by highly concentrated fertilizers such as anhydrous ammonia.
The chemistry of organic materials and environmental conditions determines:
- how rapidly organic matter is broken down,
- which soil organisms are involved in the decomposition process, and
- whether nutrient availability will increase or decrease in the short term.
|
As discussed previously, organic materials that are old or woody, such as tree branches, old roots, or dried grass, contain a large amount of carbon compared to nitrogen. To decompose these materials, soil organisms may need to extract nitrogen from the soil solution in order to balance their carbon-rich diet, thus temporarily reducing the amount of nitrogen available for plant use. Young, succulent, "first-growth" plant materials, fresh manure, and materials that have gone through primary decomposition processes by larger soil organisms contain a higher concentration of nitrogen in relation to carbon. Soil organisms more readily decompose these materials and make nutrients in them available for plant uptake.
The type of organic matter being decomposed will also affect the type of soil organisms involved in the decomposition process. As each decomposer feeds, it uses some nutrients for its own growth and reproduction and releases other nutrients into the soil solution where they are available for plant growth and production. Decomposer organisms may also excrete organic materials that can either be further broken down by other soil organisms or become part of the soil humus.
In general, bacteria require more nitrogen in order to break down organic matter than do most fungi. Fungi are the dominant decomposer in forest environments since they require less nitrogen in their diet and are able to feed on woody, older, or more fibrous materials. They are also able to survive and replicate in environments that are less moist than those required by bacteria. Bacteria are more prevalent in garden and pasture environments because they require higher amounts of nitrogen, more moisture, and feed readily on fresh manure, young grasses, legumes and other easy-to-decompose materials.(10)
Back to top
Primary Decomposers
Earthworms are primary decomposers of leaf litter and manure piles. Research conducted in Denmark showed that earthworms were responsible for 50% of the breakdown and disappearance of cow manure, while dung beetle larvae accounted for between 14 and 20%.(76) These organisms also consume fresh organic materials, then deposit their feces in the soil. When they burrow, they move manure and other organic materials into the soil, where it is more accessible to other organisms involved in decomposition. Burrowing organisms also aerate the soil. Good aeration promotes the growth of the majority of organisms involved in organic matter decomposition. For this reason, earthworms and dung beetles are visible indicators of soil health: their presence shows that nutrient decomposition processes are occurring and the soil food web is effectively operating.
Earthworms and dung beetles are visible indicators of soil health: their presence shows that nutrient decomposition processes are occurring and the soil food web is effectively operating. |
Earthworms
According to research studies, the weight of earthworms in the soil is directly related to pasture productivity.(77) In healthy soils with abundant earthworms, these organisms consume between 65-80 tons of manure per acre per year.(39) Earthworms also break down pasture thatch and incorporate organic matter from the thatch into the soil. Where few or no earthworms are present, pastures develop a thick thatch layer, slow rates of organic matter decomposition, and a poor crumb structure.(39)
Decomposition of organic matter by earthworms speeds up the breakdown and release of plant nutrients, particularly nitrogen and phosphorus. Earthworms consume low-nitrogen plant materials as well as high-nitrogen manure.(39) Under pasture conditions, earthworms have been shown to mineralize 10 pounds/acre/year of phosphorus in their casts.(5) Earthworms also facilitate the transformation of straw and leaf litter into soil humus.(78) The earthworm gut combines decomposed organic matter with particles of mineral soil and microorganisms, forming soil aggregates and humus-coated soil minerals.
Through their feeding and burrowing activities, earthworms
- Break down large residues
- Produce nutrient-rich casts
- Move organic matter through the soil
- Enhance soil aeration, water infiltration, and soil structure
- Improve root growth
|
Through their feeding and burrowing activities, earthworms move organic matter through the soil, enhancing soil aeration, water infiltration, and soil structure. They also improve root growth by creating channels lined with nutrients (79) and help till the soil. They can completely mix the top six inches of a humid grassland soil in 10 to 20 years.(80)
Factors that contribute to an abundant population of earthworms include inputs of fresh organic matter, a medium-textured-soil, thick topsoil, a near-neutral pH, moist but well-aerated soils, and moderate temperatures. Tillage, acid-producing fertilizers, insecticides, and poorly-drained soils inhibit earthworm survival.(79)
Dung beetles
Dung beetles improve nutrient cycling, enhance soil aeration, and improve forage growth while feeding on manure and using it to provide housing and food for their young. Adult dung beetles are drawn to manure by odor. They use the liquid contents for nourishment and the roughage to form a brood ball in which the female lays a single egg. This brood ball is buried in the soil where the larva grows, eating about 40 to 50% of the interior contents of the ball while depositing its own excrement. After the larva emerges, secondary decomposers readily break down the remaining dung ball.(81)
An adequate population and mix of dung beetle species can remove a complete dung pile from the soil surface within 24 hours. |
An adequate population and mix of dung beetle species can remove a complete dung pile from the soil surface within 24 hours.(82) This process decreases the potential for ammonia volatilization and nutrient runoff while making manure nutrients available to secondary decomposers within the soil profile. While moving dung into the soil, dung beetles create tunnels that enhance soil aeration and water infiltration. Dung removal also increases forage availability, since it minimizes the amount of forages that animals would avoid if dung were present.
Environmental conditions that enhance activities of dung beetles include adequate soil moisture levels and warm temperatures. Dung beetle larvae are susceptible to some insecticides used for fly and internal parasite control for cattle. Both injectable and pour-on formulations of Ivermectin (Ivomec and Doramectin), applied to cattle at the recommended dosages, reduced survival of the larvae for 1-3 weeks. However, when administered as a bolus, effects on dung beetle populations lasted up to 20 weeks.(83)
Back to top
Secondary Decomposers
Soil organisms are responsible not only for the mineralization and release of nutrients from organic material; they are also important for retaining nutrients in the soil, improving soil structure through the formation of aggregates and humus, degrading toxic substances, and suppressing diseases. Nutrients held in the bodies of soil organisms gradually become available for plant uptake and meanwhile they are protected against being lost through leaching, runoff, or other processes. Soil organisms involved in nutrient cycling release nutrients as they defecate and die. While they are still alive, these organisms conserve nutrients within their bodies.
Soil microorganisms are responsible for
- nutrient mineralization
- retaining nutrients in the soil
- forming aggregates
- degrading toxic substances
- suppressing diseases
|
Bacteria and Fungi
Bacteria and fungi are the most prevalent soil organisms. Bacterial decomposers feed on root exudates as well as on plant litter and manure. Maintaining actively growing soil roots provides a nutrient rich habitat for the growth of many bacterial species. Some species of bacteria are able to detoxify pollutants while other species, particularly rhizobia and bluegreen algae, are able to fix nitrogen. Bacterial gels are an important component of soil aggregates. Fungi decompose complex or more carbon-rich forms of organic matter. They also form soil aggregates by binding them with fungal threads or hyphae. Mycorrhizal fungi enhance nutrient and water uptake by plants by extending the length and surface area of root uptake.
In dry rangelands, crusts composed of green algae, bluegreen algae, lichens, fungi, and bacteria form over the soil surface. These crusts provide surface cover, erosion control, and soil aggregation. They are also involved in nitrogen fixation and nutrient decomposition. Crust organisms are most active during the cooler, moister part of the year when plant cover is minimal.(2)
Amoebas, nematodes, and protozoa
Amoebas, nematodes, and protozoa feed on bacteria and fungi. Protozoa feeding on bacteria stimulate their growth and aggregate forming activities.(78) Nematodes may consume up to 25% of the bacteria in the soil.(84) According to one study, nematodes feeding on bacteria accelerated litter decomposition by 23%.(85) Both protozoa and nematodes release nutrients to the soil system, making them available to plants and other soil organisms.
Mutualistic relationships
In undisturbed ecosystems, plants and soil organisms have co-evolved to form mutualistic relationships. Plants provide carbohydrates and other nutrient-rich substances through their root system, providing an excellent source of food for soil organisms. As a result, populations of soil organisms involved in nutrient decomposition are greatest next to plant roots.(85) These organisms provide plants with nutrients necessary for their growth, produce hormones and other chemicals that improve plant vigor, and protect the plant against diseases. When the need of plants for nutrients is low, soil organisms will hold nutrients in their bodies rather than release them into the soil solution.(10) This mutualistic relationship is disturbed during plowing, cultivation, and harvesting. When plant roots are removed, populations of soil organisms decrease since they no longer have a source of nourishment and habitat.
Back to top
Soil Organisms and Soil Health
Soil health refers to the ability of soils to function as a productive environment for plant growth, an effective filter, and an efficient regulator of water flow. Soil mineralogy and chemistry form the basis for soil composition and soil health. However, much of soil health and function depends on an active community of diverse soil organisms. Nutrient cycling, aggregate formation, degradation of toxins, creation of soil pores, and absorption of water and nutrients are all functions of soil organisms.
The activities of soil organisms serve as effective indicators of current land productivity and its ability to withstand degradation. By monitoring these indicators, farmers, soil conservationists, and other land managers can implement appropriate practices to minimize soil or nutrient losses, enhance nutrient cycling, and increase plant productivity. The Soil Quality Institute has taken the lead in developing and promoting the use of soil health indicators.(86) Qualitative, "farm-based" and "farmer friendly" indicators are incorporated into soil health cards specific to location and farming practice.(87) These cards can be used to monitor the relative health and productivity of soils, identify areas of concern, and enhance awareness of the relationships between soil health and crop production. Below is a soil health card for pastures based on a compilation of indicators from soil health cards developed in various locations.
Table 15: Pasture Soil Health Card |
Indicator |
Good |
Medium |
Poor |
Pasture cover |
Complete cover of forages and litter over entire pasture |
Limited bare patches. No extensive bare areas near drainage areas |
Extensive bare patches especially near watering or other congregation areas |
Plant diversity |
Diversity of plant species, including forbs, legumes, and grasses, and differences in leaf and root growth habits. |
Limited number of species and diversity of growth habit. Some invasive plants present. |
Less than three different species, or invasive species are major components of the plant mix. |
Plant roots |
Abundant vertical and horizontal roots |
More horizontal roots than vertical |
Few roots, most are horizontal |
Soil life— macroorganisms |
Many dung beetles and earthworms present |
Few dung beetles and earthworms present |
No dung beetles or earthworms present |
Soil compaction |
Wire flag enters soil easily. Does not encounter hardened area at depth |
Can push wire flag into soil with difficulty or encounters hardened area at depth |
Cannot push wire flag into soil |
Erosion |
No gullies present, water running off pasture is clear |
Small rivulets presents, water running off pasture somewhat muddy |
Gullies present, water running off pasture is very muddy |
Soil aggregation |
Soil in clumps, holds together when swirled in water |
Soil breaks apart after gentle swirling in water |
Soil breaks apart within one minute after being in water |
Water infiltration |
Water soaks in during moderate rain, little runoff or water ponding on soil surface. |
Some runoff during moderate rainfall, some ponding on soil surface |
Significant runoff during moderate rainfall, much water ponding on soil surface |
Adapted from the Georgia, Mon-Dak, and Pennsylvania Soil Health Cards (86) Sullivan (88) and USDA (89). |
Table 16: Soil Organisms |
Bacteria—the most numerous microorganism in the soil. Every gram of soil contains at least a million of these tiny one-celled organisms. Decompose simple or nitrogen-rich organic matter. Require moist environments. Also responsible for nitrogen fixation, soil aggregate formation, and detoxification of pollutants. |
Actinomycetes—thread-like bacteria, which look like fungi. They are decomposers and responsible for that sweet, earthy smell of biologically active soil. |
Fungi—multicelluar microorganisms that usually have a thread-like structure. Mycorrhizae form extensions on roots, increasing their ability to take up nutrients and water. They also transport nitrogen from legumes to grasses. Yeasts, slime molds, and mushrooms are other species of fungi. |
Algae—microorganisms that are able to make their own food through photosynthesis. They often appear as a greenish film on the soil surface following a rainfall. |
Protozoa—free-living animals that crawl or swim in the water between soil particles. Many soil protozoa species are predatory and eat other microorganisms. By feeding on bacteria they stimulate growth and multiplication of bacteria and the formation of gels that produce soil aggregates. |
Nematodes—small, wormlike organisms that are abundant in most soils. Most nematodes help decompose organic matter. Some nematodes are predators on plant-disease-causing fungi. A few species of nematodes form parasitic galls on plant roots or stems, causing plant diseases. |
Earthworms—multi-cellular organisms that decompose and move organic matter through the soil. Earthworms thrive where there is little or no tillage, especially in the spring and fall, which are their most active periods. They prefer a near-neutral pH, moist soil conditions, an abundance of plant residues, and low light conditions. |
Other species of soil organisms—Many other organisms, including dung beetles, sowbugs, millipedes, centipedes, mites, slugs, snails, springtails, ants, and birds, facilitate nutrient cycling. They make residues more available to smaller organisms by breaking them down physically and chemically and by burying them in the soil. |
Table 17: Pasture Management Practices to Maintain a Healthy Soil Food Web
Provide soil organisms with a balanced diet
- Manure and perennial pastures provide food for soil organisms
- Succulent materials that are more nitrogen-rich are more rapidly decomposed than materials that are older, woodier and contain less nitrogen.
Provide soil organisms with a favorable environment
- Most beneficial soil organisms prefer a well-aerated environment
- Decomposer bacteria generally prefer an environment that is moist, has a near neutral pH, and has easy-to-decompose materials
- Decomposer fungi generally prefer an environment that is acid, moderately dry, and has more carbon-rich, complex organic materials
- Continuous plant growth maintains environment of actively growing roots in the soil. The root or rhizosphere environment is a very nutrient rich habitat for the growth of many soil organisms.
Use practices that favor the growth of soil organisms
- Maintain a balance between intense grazing and adequate rest or fallow time
- Encourage movement of grazing animals across pastures to feed and distribute manure evenly as well as to kick and trample manure piles
- Maintain a diversity of forage species to provide a variety of food sources and habitats for a diversity of soil organisms.
Avoid practices that kill or destroy the habitat of soil organisms
- Avoid the use of Ivomectin deworming medications, soil-applied insecticides, and concentrated fertilizers such as anhydrous ammonia and superphosphate
- Minimize tillage and cultivation practices
- Minimize practices that compact the soil, such as extended grazing practices or grazing wet soils.
|
Back to top
Chapter 5: Pasture Management and Water Quality
Effective nutrient cycling and judicious applications of fertilizers and manure enhance plant growth. However, if nutrients are applied at the wrong time or in excess of what plants can use, they increase the potential contamination of nearby rivers and lakes. Similarly, grazing practices can degrade water quality if grazing intensity is too great, if paddocks are used when the soil is too wet, or if the duration of rest periods is too short. Long-term intensive grazing practices can negatively affect water quality, especially when combined with heavy fertilization with either mineral or manure nutrients. Likely impacts include contamination of groundwater with nitrates and contamination of surface water with phosphate, sediments, and pathogens.(90, 91)
Risk Factors for Nutrient Losses
Nutrient loss pathways
If more manure or fertilizer nutrients are applied to pastures than are used in the growth of forage crops, excess nutrients will either accumulate in the soil or be lost through leaching, runoff, or erosion. Nutrient accumulation occurs when minerals in the soil have the ability to bind or hold particular nutrients. Sandy or silty soils or soils with a near-neutral pH do not bind phosphorus well. When more phosphorus is applied to these soils than is used for plant growth, the excess phosphorus can be easily dissolved and carried away by runoff water to lakes and streams. Both acid clay soils and soils with a high calcium carbonate content have a strong ability to bind large amounts of phosphorus. If only moderate excesses of phosphorus are applied to these soils or if excess phosphorus is applied to the soil only occasionally, these soils will be able to bind the excess phosphorus and hold it against leaching. However, if phosphorus fertilizers or manure are continuously applied at high rates, phosphorus levels will eventually build up in the soil to the extent that soils will no longer be able to hold onto the additional phosphorus and these excesses will be susceptible to loss through runoff. High levels of phosphorus in surface water cause eutrophication or algae blooms. When sources of drinking water have significant algae growth, chlorine in the water treatment process reacts with compounds in the algae to produce substances that can increase cancer risks.
Unlike phosphorus, nitrogen does not readily bind to soil minerals or organic matter. As a result, it easily leaches through the soil, especially if high rainfall follows manure or nitrogen fertilizer applications and the soil is sandy or gravelly. High levels of nitrate in ground water used for drinking can cause health problems for human babies and immature animals. Management practices that minimize the potential for nitrogen leaching include not applying excess levels of nitrogen and avoiding manure or nitrogen fertilizer applications during times when plants are not actively growing.
Erosion occurs when water or wind moves soil particles, resulting in the loss of topsoil and of the nutrients, toxins, and pathogens attached to these particles. Erosion by water can also transport surface-applied manure into lakes, rivers, and streams. Water quality concerns associated with erosion include siltation, fish kills, eutrophication, and degraded quality for recreational and drinking water uses.
Nutrient balances
Water contamination problems associated with farming are becoming an increasing societal and political concern. The Federal Clean Water Act mandates states to minimize non-point source pollution or pollution associated with runoff and erosion, much of this originating from agricultural lands.(92) Currently, water quality regulations are primarily focused on larger farms that have a high concentration of animals and use high inputs of purchased feeds. Societal concerns about farming operations are increasing as increasing numbers of non-farm families move into rural areas and urban growth decreases the distance between farm and non-farm community members.
On farms that have a high numbers of animals, a limited land area, and high use of feeds that are not grown on the farm, nutrient imbalances exist. This is because the amount of nutrients that accumulate in the animal manure is greater than the needs of all crops being grown on the farm. Maintaining a balance between the amount of nutrients added to the soil as manure and fertilizer and the amount of nutrients removed as forages, hay, crops, or livestock is critical for productive crop growth and water quality protection. If more nutrients are removed than are returned to the system, crop production will decline. If more nutrients are added than can be used for productive crop growth, nutrients will build up in the soil, creating a high risk for leaching, runoff, and water contamination.
While large farms are primarily being targeted by environmental regulations, these farms are not the only livestock operations at risk for contaminating water quality. Often, smaller livestock farms pose more risk than larger operations. For instance, on smaller dairy farms the barn is commonly located near a stream because it was built prior to rural electrification and the ability to pump water from wells or streams to watering troughs. On various small livestock operations, animals have access to paddocks located near a well head or over highly permeable soils because land area is limited. Riparian areas are less likely to be protected by fencing or buffer areas. On farms without adequate manure storage facilities, manure is often applied to poorly drained or frozen fields during the winter, resulting in a high potential for surface water contamination. In addition, on smaller farms, necessary equipment or labor is often not available to properly apply manure according to a nutrient management plan. Careful management of animal grazing and manure handling practices are critical on all farms in order to protect water resources.
Manure or fertilizers should be applied when the nutrients in these materials can be most effectively used for plant growth and production, and never to ground that is snow-covered, frozen, or saturated. |
Back to top
Pathogens
Pathogens in manure
Although not directly related to nutrient cycling, pathogens are a critical water quality concern associated with manure management. Animal grazing or manure applications can contaminate water bodies not only with excess nutrients but also through parasites in feces. Parasites of greatest concern are E. coli, Giardia, and Cryptosporidium. E. coli is of most concern to rural residents dependent on well water and of limited concern to public water users since this parasite is killed by municipal water purification and treatment processes. Typically, this parasite causes mild to moderate gastrointestinal problems. However, new strains of E. coli have killed people who are very young, very old, or have weakened immune systems. Giardia and Cryptospordium are pathogens with a dormant stage that is very resistant to purification treatment. Almost all municipal water treatment facilities are required to use secondary filtration processes that remove these resistant forms from the water supply. Most private wells, however, do not the capability of filtering out these pathogens. Like the virulent strain of E. coli, Giardia and Cryptospordium cause gastrointestinal problems that can be fatal for people with weak or undeveloped immune systems.
Minimizing the risk of pathogen movement into water bodies involves ensuring that animals, especially young calves, are not exposed to or kept in conditions that make them susceptible to these diseases. Any manure that potentially contains pathogens should either be completely composted before being land applied or applied to land far from streams and at low risk of erosion or runoff.(93)
Pasture management practices to reduce risks of pathogen contamination
Manure or fertilizers should be applied when the nutrients in these materials can be most effectively used for plant growth and production, and never to ground that is snow-covered, frozen, or saturated. Under such wet or frozen conditions, manure or fertilizer nutrients are not bound by soil particles. Instead, these nutrients are lying unbound on the soil surface where they have a high potential to be carried away by runoff into lakes or streams. Pathogens in manure applied to frozen or snow-covered soil will not be in contact with other soil organisms. In addition, most predatory soil organisms will be in a dormant state and unable to decrease pathogen numbers before snowmelts or heavy rainfalls cause runoff. Areas that need to be protected from contamination by nutrients and parasites in animal feces include well heads, depressions at the base of hills, drainage ways, rivers, streams, and lakes. Well heads and water bodies need protection because they serve as drinking and recreational water sources, while foot slopes and drainage ways have a high potential for nutrient runoff and transport of contaminants to water bodies.
Back to top
Nitrate Contamination
Nitrate is not held by soil particles and is easily leached, especially through porous soils, such as sandy soils or soils with cracks or fissures that allow for rapid movement of excess nitrogen through the soil profile. Where excess nitrogen is not applied, nitrate leaching in pastures is minimal. High nitrate leaching losses were observed, however, when orchard grass pastures in Pennsylvania were fertilized with 200 pounds/acre of nitrogen as ammonium nitrate.(45) These researchers also calculated that a stocking rate for Holstein dairy cows of 200 animal days would result in nitrate leaching from urine in excess of drinking water standards (10 mg/liter).(45) In pastures where nitrogen was provided by nitrogen-fixing legumes, nitrate leaching was minimal when environmental conditions were normal. But high nitrate leaching was observed when a severe drought followed good growing conditions, causing legume nodules to die and release nitrogen into the soil.(20)
Nitrate concentrations greater than 10 ppm in well water may cause nitrate toxicity or methemoglobinemia. This ailment which affects infant children as well as young chickens and pigs, and both infant and adult sheep, cattle, and horses, increases nitrate concentration in the bloodstream and prevents the uptake and use of oxygen, thus causing suffocation. Pregnant animals that are affected may recover then abort within a few days.(21) Personnel associated with local health departments can test wells to determine nitrate concentrations.
Back to top
Phosphorus Contamination
Phosphorus can be transported from fields or pastures into lakes or streams either as a component of erosion or within runoff water. Phosphorus that is dissolved in runoff water has a greater effect on water quality than phosphorus that is attached to soil particles transported to water bodies by erosion.(94) This is because the dissolved phosphorus is more available for use by algae and other aquatic organisms that cause eutrophication, noxious greening of water bodies, and fish kills. Phosphorus associated with soil particles tends to settle to the lake or river bottom where it remains biologically stable or only slowly available for use by aquatic organisms.
Dissolved phosphorus
In pastures, sources of dissolved phosphorus include manure or phosphorus fertilizers lying on the soil surface, and wet soils that have a high phosphorus concentration. Runoff water can readily dissolve soluble phosphorus in manure or phosphorus fertilizers. When the amount of phosphorus in soil exceeds the ability of soil particles to bind onto it, the excess phosphorus can readily be dissolved and transported by runoff water, especially when soils are saturated. Dissolved phosphorus has the greatest potential for being transported from pastures into waterbodies when rainfall is heavy, when high levels of phosphorus are present either on the surface of the soil or within the soil, and when pastures are located within 350 feet of waterbodies.(95)
Increasing forage diversity generally decreases runoff potential. Care should be taken to combine species, such as bunchgrasses, that enhance water infiltration but expose the soil surface between clumps,(96) with closer-growing species such as tall fescue or prostrate species such as white clover. A combination of native forages and low-growing, shade-tolerant plants could allow for both enhanced water infiltration and cattle production (Brauer, personal communication). Setting up paddocks on the contour can also allow downslope paddocks that are regenerating following grazing to serve as buffer strips for upslope paddocks that are currently being grazed. Potential runoff from manure can also be reduced by applying it to alternate paddocks set up as contour strips (D.E. Carman, personal communication).
Phosphorus associated with erosion
Soil-attached phosphorus can be transported to water bodies by erosion. Low-level sheet erosion contributes more phosphorus than higher-impact rill or gully erosion. This is because sheet erosion primarily transports nutrient-enriched topsoil, manure, and plant residues while gully erosion transports more nutrient-poor subsoil.(27) As with runoff, the amount of phosphorus transported by erosion is greatest during intense rainfalls or snowmelts. Pasture soils that are completely covered by vegetation are protected against the forces of erosion. Erosion occurs primarily when soils are bare and land is sloping.
Impact of phosphorus contamination on water quality
The impact of phosphorus runoff on stream or lake water quality is greatest during summer and fall. While spring rains or snowmelts may transport a greater total amount of phosphorus, the large amount of water in the runoff dilutes the phosphorus so that it is in a relatively low concentration when it reaches water bodies. In contrast, intense rains falling on soils and pastures during the summer are likely to run off rather than soaking into dry, hard soils. When intense rains fall on pastures with surface-applied manure or phosphorus fertilizers, runoff water will carry a high concentration of dissolved phosphorus into streams. If these streams have relatively low water flows, the runoff water will create a high concentration of phosphorus in streams, which then causes algae and other nuisance plants to grow.(27)
Table 18. Impacts of excess phosphorus on water quality
- Excessive phosphorus stimulates growth of algae and aquatic weeds in lakes and streams
- Rapid algal and aquatic-weed growth depletes oxygen from water, leading to death of fish
- Outbreaks of certain aquatic organisms dependent on high phosphorus levels can cause health problems in humans, livestock, and other animals
- When water that has high algal growth is chlorinated for use as drinking water, carcinogenic substances are formed
|
The type of phosphorus fertilizer used influences the potential risk of water contamination. Highly soluble fertilizers such as superphosphate present a greater short-term potential for phosphorus loss since it is easily dissolved and transported. In the long term, however, less- soluble fertilizers, such as dicalcium phosphate, may pose a greater risk. This is because less- soluble fertilizer remains on the soil surface and available for dissolution and runoff for a longer time.(27) Impacts on water quality from sediment-attached phosphorus fertilizer have been observed to persist for up to six months.(97) Runoff risks can be substantially decreased if fertilizers are incorporated into soil and applied according to a nutrient management plan.
Phosphorus index
The phosphorus index was developed to address federal and state water quality guidelines while recognizing that phosphorus movement is influenced by local environmental conditions and land management practices. Each state is developing its own phosphorus index to ensure that it is appropriate to local conditions. Each phosphorus index contains a component related to phosphorus sources, soil test phosphorus, manure phosphorus, and fertilizer phosphorus. Soil test phosphorus accounts for the plant available or soluble phosphorus in the soil, derived either from the mineral base of the soil or from decomposing organic matter. The potential for manure or fertilizer phosphorus to be lost through runoff depends on the amount applied, how it was applied, and when it was applied. Manure and fertilizer that is incorporated into the soil at rates required for crop growth during or just prior to the time of crop production poses minimal risk to water quality. Conversely, surface-applying excess amounts of manure or fertilizer when crops are not actively growing or when the soil is saturated, frozen, or snow-covered will pose high risks for phosphorus run off. However, a high concentration of phosphorus in the soil or applied to the soil will not pose a risk to water quality unless there is a means of transporting this phosphorus to water bodies. Methods for transporting phosphorus from farm fields to water bodies include erosion, runoff, and flooding. Locations that have a high source of phosphorus and a high risk of transport are critical source areas or locations where management practices need to carefully consider risks of phosphorus losses.
Figure 14. Phosphorus Index Components

|
Back to top
Subsurface Drainage
Artificial subsurface drainage makes normally wet soils drier, and decreases the wet period for seasonally wet soils, by allowing more water to seep into the soil profile. Subsurface drainage has been shown to decrease water runoff by 72% and total phosphorus losses due to runoff by 50%.(5)
Contaminant transport through drains
Unfortunately, the advantage that subsurface drainage provides in decreasing runoff potential may be overshadowed by the ability of drainage systems to directly transport nutrients from fields to waterways. Most subsurface drainage systems were installed primarily for production reasons: to allow farmers to work their fields earlier in the year and to minimize plant stunting and disease problems associated with saturated soils. Since many of these systems were installed before agricultural impacts on water quality became a societal concern, agricultural drains often empty directly into streams and rivers.
Because artificial drainage makes fields drier, farmers can drive tractors or other equipment onto these fields earlier in the year. Farmers who have a full manure-storage system to empty, or who have time constraints for spreading manure in advance of planting, may be tempted to apply manure and fertilizers to these fields during times when the soil would be wet if it was not drained. Farmers with a limited land base may want to graze these fields when the weather is wet. However, to protect water quality, these fields should be managed as though artificial drainage had not been installed. Nutrients in fertilizers or manure can leach through the soil to drainage pipes. If artificially drained fields are used for nutrient applications or grazing while water is flowing out of drainage outlets, drainage water can carry these leached nutrients directly to streams or rivers.
In soils with subsurface drainage, cracks and channels provide a direct pathway for nitrate, phosphorus, or soluble manure to move from the soil surface to subsurface drains.(6, 78) Because these channels are relatively large, contaminants are not absorbed by soil particles or biologically treated by soil organisms.(27) Soil cracks or channels develop through earthworm burrowing, death and decomposition of taproots, and soil drying. The direct connection of cracks or channels in soils to artificial drainage pipes can transport phosphorus and pathogens from manure applications directly to drainage outlets usually within an hour after the onset of a heavy rain.(6) Another research study showed that a single rotational grazing event doubled the amount of sediment and increased the amount of dissolved phosphorus in tile drainage water 15-fold compared to an ungrazed site.(5)
Management practices
To minimize the potential for water contamination, land that is artificially drained should not be grazed or have fertilizer or manure applied during times when drainage water is flowing from the field or just prior to a rainstorm. Alternatively, contaminated water flowing out of tile drains should not be allowed to empty directly into rivers or streams. Instead, it should be directed to a grassed filter or buffer area or treated in a wetlands area where biological and chemical processes lower contaminant levels through sedimentation and absorption.(27)
Back to top
Riparian Buffers
A well-designed buffer with a combination of trees, shrubs, and herbaceous plants has the ability to trap sediments and nutrients associated with these sediments. Buffers also provide habitat for riverbank and aquatic animals. An effective buffer for trapping sediments contains a combination of grasses and herbaceous plants that are able to catch sediments in their foliage or residues. The root channels around actively growing plants will also absorb slow-moving runoff water and plants in the buffer area will use transported nutrients for their growth. Regular harvest and removal of buffer vegetation can delay or prevent the buildup of nutrients in the buffer area. However, harvests must be conducted in a manner that does not decrease the ability of buffers to capture sediments and absorb runoff water.
Riparian buffers are limited in their ability to remove soluble phosphorus and nitrate from runoff water, especially if flows are intense.(98) During heavy rainstorms or rapid snowmelts, buffers generally have limited effectiveness for controlling the movement of runoff-borne nutrients from being transported into water bodies. This is because water from these heavy flows concentrates into rapidly moving channels that can flow over or through buffer areas.
The continual transport of phosphorus-rich sediments into buffers will cause a buildup of high concentrations of phosphorus in buffer areas. Eventually, these areas will lose their ability to hold additional phosphorus and the buffer areas can become a source for phosphorus entering water bodies rather than an area that captures phosphorus before it enters water bodies.(99)
Back to top
Riparian Grazing
When grazing animals have continuous, unlimited access to riparian areas, their activities break down stream banks, alter stream flow, cause decreased vigor of stream bank vegetation, and diminish the species and populations of fish and aquatic wildlife.(100, 101) Cattle grazing in riparian areas trample on streamside and aquatic organisms, disturb the habitat of these organisms, decrease oxygen availability by suspending bottom sediments, and contaminate streams by directly depositing manure and urine.(102) Animal movement along streambanks or within streams also contributes to bank erosion. In addition, grazing activities alter the amount and type of plant residues available for the growth and reproduction of riparian organisms.(90)
Managed grazing of riparian areas can protect water quality and improve riparian habitat. In Wisconsin, researchers studying intensive rotational grazing practices restricted livestock access to riparian areas to 5-20 days per season. Limiting animal access to riparian areas allows a thick vegetative turf to develop throughout the paddock, which stabilized stream banks and reduced streambank erosion.(102) For dairy cattle each grazing period should last only 12 to 24 hours, while beef cattle and sheep can be grazed for 3 to 4 days each time.(103) Grazing should not be allowed to reduce herbage stubble to less than 4 to 6 inches in height. This will protect water quality by providing adequate plant growth, bank protection and sediment entrapment.(104)
Pasture management practices should discourage animals from congregating in the stream or on the streambank, where their manure can pollute these water bodies. Shade, salt licks, and other sources of supplemental nutrients should be located at least 15 feet from the stream bank to provide a buffer between areas of manure deposition and the stream.(100)
The season in which riparian areas are grazed is also an important consideration if water quality is to be protected. Grazing in the spring or early summer followed by complete livestock removal in the summer allows riparian plant regrowth to occur before the dormant period in the fall. Animals will damage streambanks if they are allowed to graze riparian areas in the winter when soils are freezing and thawing or in the spring when soils are wet. During drought conditions, streambanks should not be grazed since vegetation will be slow to recover. In addition, animals should not be allowed to graze riparian areas in the summer when hot, dry conditions will encourage cattle to congregate in the water.(103) Streambanks that have a high soil moisture content, a fine soil texture, or that are prone to erosion, are subject to early-season grazing damage and should not be grazed in the spring or not until they have dried.(104) Use of floating fences and graveled access areas can control animal access to water, minimizing the impacts on streambank stability and ecology.
Back to top
Table 19.
Pasture Management Practices to Protect Water Quality
Minimize congregation of animals in pastures
- Use practices that encourage the movement of animals across paddocks
- Avoid overgrazing of pastures
Minimize the potential for nitrate leaching
- Encourage animal movement across paddocks
- Maintain a healthy cover of actively growing forages across paddocks
- Rotate pastures to maximize nutrient uptake by plants
Minimize the potential for nutrient runoff
- Do not apply fertilizers or manure to saturated, snow-covered, or frozen ground
- If possible, compost manure before applying it to soil. This will minimize pathogen populations while transforming nutrients into more stable compounds
- Do not use pastures that are wet, flooded, or saturated
- Use practices that favor populations of soil organisms that rapidly incorporate manure into the soil
- During cold or wet weather, do not use pastures that are located next to a river, stream, or waterway
- Recognize that buffers are not effective in controlling the movement of nutrients carried by runoff water, especially when flows are intense
Minimize the potential for erosion
- Maintain a complete cover of forages and residues across the surface of all paddocks
- Use practices that minimize the congregation of animals or the repeated trampling of animals on the same lounging area or pathway
- Riparian areas should only be grazed using short-term intensive grazing practices, and then only during spring and early summer
- Maintain riparian buffers (including a combination of herbaceous plants, tress, and shrubs) adjacent to rivers, streams, and lakes to act as a filter for eroded soil and other contaminants
Minimize water contamination from artificial drainage systems
- During wet weather, do not use pastures that are on artificially-drained land
- Modify outlets from drainage ways to treat drainage water in wetlands or on filter areas before it flows into streams or other water bodies
|
References
1. Magdoff, F. and H. van Es. 2000. Building Soils for Better Crops, Second Edition. Sustainable Agriculture Network. Handbook Series Book 4. Beltsville, MD.
2. Belnap, J., H.H. Kaltenecker, R. Rosentreter, J. Williams, S. Leonard, and D. Eldridge. 2001. Biological Soil Crusts: Ecology and Management. Technical Reference 1730-2. United States Department of the Interior. Bureau of Land Management. Denver, CO. Accessed at:
www.blm.gov:80/nstc/library/techref.htm.
3. Welch, T.G., R.W. Knight, D. Caudle, A. Garza, and J.M. Sweeten. 1991. Impact of Grazing Management on Nonpoint Source Pollution. Texas Agricultural Extension Service. Extension Resource Center. Document L-5002. Accessed at: <http://texaserc.tamu.edu/catalog/query.cgi?id=433> [link no longer active].
4. Pellant, M., P. Shaver, D.A. Pyke, and J.E. Herrick. 2000. Interpreting Indicators of Rangeland Health. Version 3. Technical Reference 1734-6. United States Department of Agriculture. Bureau of Land Management. National Science and Technology Center Information and Communications Group. Denver, CO. Accessed at: www.blm.gov/nstc/library/pdf/1734-6.pdf.
5. Sharpley, A.N. and J.K. Syers. 1979. Loss of nitrogen and phosphorus in tile drainage as influenced by urea application and grazing animals. New Zealand Journal of Agricultural Research. Volume 22. p. 127-131.
6. van Es, H. and L. Geohring. 1993. Rapid movement of manure to tile drainage lines: Should we be concerned? What's Cropping Up? Vol. 3. No. 4. p. 5, 7.
7. Hillel, D. 1998. Environmental Soil Physics. Academic Press. San Diego, CA.
8. Thurow, T.L., W.H. Blackburn, and C.A. Taylor, Jr. 1988. Infitration and interrill erosion responses to selected livestock grazing stategies, Edwards Plateau, TX. Journal of Range Management. Vol. 41. p. 296-302.
9. Pearson, C.J. and R.L. Ison. 1987. Agronomy of Grassland Systems. Cambridge University Press. Cambridge. p. 62-78.
10. Ingham, E. 1996. The soil foodweb: Its importance in ecosystem health. Home page. Accessed at: http://rain.org:80/~sals/ingham.html.
11. Whitehead, D.C. 2000. Nutrient Elements in Grassland Soil-Plant-Animal Relationships. CAB International Publishing. Wallingford, Oxon, UK.
12. Woodmansee, R.G., I. Vallis, and J.J. Mott. 1981. Grassland Nitrogen. In: F.E. Clark and T. Rosswall. (eds.) Terrestrial Nitrogen Cycles: Processes, Ecosystem Strategies and Management Impacts. Ecological Bulletins. Stockholm. Vol. 33. p. 443-462.
13. Joost, R. 1996. Pasture Soil Fertility Management. p. 35-46. In: J. Gerrish, and C. Roberts (eds.) 1996 Missouri Grazing Manual. University of Missouri. Columbia, MO.
14. West, C.P. and A.P. Mallarino. 1996. Nitrogen transfer from legumes to grasses. In: R.E. Joost and C.A. Roberts (eds.) Nutrient Cycling in Forage Systems. Potash and Phosphate Institute and Foundation of Agronomic Research. Manhattan, KS.
15. Brophy, L.S., G.H. Hiechel, and M.P. Russelle. 1987. Nitrogen transfer from forage legumes to grasses in a systematic planting design. Crop Science. Vol. 27. p. 753-758.
16. Thomas, R.J. 1992. The role of the legume in the nitrogen cycle of productive and sustainable pastures. Grass Forage Science. Vol. 47. p. 133-142.
17. Russelle, M.P. 1992. Nitrogen cycling in pasture and range. Journal of Production Agriculture. Vol. 5 No. 1 p. 13-23.
18. Clough, T.J., R.R. Sherlock, K.C. Carmeron, and S.F. Ledgard. 1996. Fate of urine nitrogen on mineral and peat soils in New Zealand. Plant and Soil. Vol. 178. p. 141-152.
19. Williams, P.H. and R.J. Haynes. 1994. Comparison of initial wetting pattern, nutrient concentrations in soil solution and the fate of 15N-labelled urine in sheep and cattle urine patch areas of pasture soil. Plant and Soil. Vol. 162. p. 49-59.
20. Stout, W.L., S.L. Fales, L.D. Muller, R.R. Schnabel, and S.R. Weaver. 2000a. Water quality implications of nitrate leaching from intensively grazed pasture swards in the northeast US. Agriculture, Ecosystems, and Environment. Vol. 77. p. 203-210.
21. Eubank, W., J.D. Carpenter, B.A. Maltberger, N. Anderson, and K. Mancl. 1998. Nitrate in Drinking Water. Water Quality Initiative publication WQ103. Available at: http://muextension.missouri.edu/explore/envqual/wq0103.htm
22. Anon. 1988. Watch out for nitrate toxicity. Wallace Farmer. August 9, 1988. p. 48.
23. Daniels, M., T. Daniel, D. Carman, R. Morgan, J. Langston, and K. VanDevender. 1998. Soil Phosphorus Levels: Concerns and Recommendations. University of Arkansas. Division of Agriculture. Cooperative Extension Service. Accessed at: www.sera17.ext.vt.edu//Documents/Soil_P_Levels_Concerns_and_Recommendations.pdf.
24. Moore, P.A. Jr. and P.R. DeLaune. 2000. Development of a phosphorous index for pastures: I. Rainfall simulation data. Proceedings of the Southern American Society of Agronomy Meeting.
25. Lemunyon, J.L. and R. G. Gilbert. 1993. The concept and need for a phosphorus assessment tool. Journal of Production Agriculture. 6:483-486.
26. Snyder, C.S., T.W. Bruulsema, A.N. Sharpley, and D.B. Beegle. 1998. Site-specific use of the environmental phosphorus index concept. Site-Specific Management Guideline-1. Potash and Phosphate Institute. Conway, AR.
27. Gillingham, A.G. and B.S. Thorrold. 2000. A review of New Zealand research measuring phosphorus in runoff from pasture. Journal of Environmental Quality. Vol. 29. p. 88-96.
28. Cherney, J.H. 1996. Potassium content of grasses for dairy cattle. What's Cropping Up? Vol. 6. No. 3. p. 6-7.
29. Albrecht, W.A. and C. Walters, Jr. (eds.). 1975. The Albrecht Papers (Vols. I-IV). Acres U.S.A., Kansas City, MO.
30. Lock, T.R., R.L. Kallenbach, D.G. Blevins, T.M. Reinbott, G.J. Bishop-Hurley, R.J. Crawford, Jr. and M.D. Massie. 2001. Fertilizing tall fescue pastures with phosphorus to prevent grass tentany in grazing beef cows. In: T. Terrill and K. Cassida (eds.) American Forage and Grassland Council Proceedings. American Grassland Council. Georgetown, TX.
31. Manning, E. 2000. Sulfur's big boost. Progressive Farmer. Vol.15. No. 11. p. 74.
32. Kallenbach, R., R. Mammen, T. Hansen. 2000. Forage crops for pasture-based dairies. In: J. Bishop-Hurley, S.A. Hamilton, and R. Kallenbach (eds.) Missouri Dairy Grazing Manual. Missouri University Extension. University of Missouri. Columbia, MO.
33. Evans, R.D. and J.R. Johansen. 1999. Microbiotic crusts and ecosystem processes. Critical Reviews in Plant Sciences. Vol. 18. No. 2. p. 183-225.
34. United States Department of Agriculture/ Natural Resources Conservation Service. 2001. Rangeland Soil Quality—Physical and Biological Soil Crusts. Soil Quality Information Sheet. Rangeland Sheet 7. Accessed at: http://soils.usda.gov/sqi/files/RSQIS7.pdf.
35. Matches, A.G. 1992. Plant response to grazing: A review. Journal of Production Agriculture. Vol. 5. p. 1-7.
36. Gerrish, J.R. 1993. Management intensive grazing: principles and techniques. In: Stockman Grass Farmer North American Grazing Conference. Jackson, MS.
37. United States Department of Agriculture/ Natural Resources Conservation Service. 2001. Rangeland Soil Quality—Compaction. Soil Quality Information Sheet. Rangeland Sheet 4. Accessed at: http://soils.usda.gov/sqi/files/RSQIS4.pdf.
38. Zinati, G.M., Y.C. Li, and H.H. Bryan. 2001. Utilization of compost increases organic carbon and its humin, humic and fulvic acid fractions in calcareous soil. Compost Science and Utilization. Vol. 9. No. 2. p. 156-162.
39. Edwards, C.A. and P.J. Bohlen. 1996. Biology and Ecology of Earthworms. Chapman & Hall, New York.
40. United States Department of Agriculture/ Natural Resources Conservation Service. 2001. Rangeland Soil Quality—Aggregate Stability. Soil Quality Information Sheet. Rangeland Sheet 3. Accessed at: http://soils.usda.gov/sqi/files/RSQIS3.pdf.
41. Haynes, R.J. and P.H. Williams. 1993. Nutrient Cycling and Soil Fertility in the Grazed Pasture Ecosystem. Academic Press Inc.
42. Lory, J. and C. Roberts. 2000. Managing nutrients in pastures to improve profitability and water quality. In: G. J. Bishop-Hurley, S.A. Hamilton, and R. Kallenbach (eds.) Missouri Dairy Grazing Manual. Missouri University Extension. University of Missouri. Columbia, MO.
43. Horne, J. 1992. Soil fertility affects pasture winter hardiness and moisture use efficiency. KCSA Newsletter. Volume 18 No. 11. p. 1.
44. Klausner, S. 1995. Nutrient management: Crop production and water quality. Cornell Cooperative Extension. Ithaca, NY.
45. Stout, W.L., S.L. Fales, L.D. Muller, R.R. Schnabel, G.F. Elwinger, and S.R. Weaver. 2000. Assessing the effect of management intensive grazing on water quality in the northeast US. Journal of Soil and Water Conservation. Vol. 55. No. 2. p. 238-243.
46. Detling, J.K., 1988. Grasslands and savannas: regulation of energy flow and nutrient cycling by herbivores. In: L. R. Pomeroy and J. J. Alberts (eds.) Concepts of Ecosystem Ecology. Springer-Verlag Inc. New York. NY.
47. Wells, K.L., C.T. Dougherty. 1997. Soil Management for Intensive Grazing. Volume 18 No. 2. 6p. Accessed at: www.uky.edu/Ag/Agronomy/Extension/ssnv/ssvl182.htm.
48. Whitehead, D.C. 1995. Nitrogen leaching from soils. In: D.C. Whitehead (ed.) Grassland Nitrogen. CAB International. Oxon. UK.
49. Dalrymple, R.L. 1994. Nutrient recycling. Agricultural News and Views. Vol. 12 No. 4. p. 3.
50. Wilkinson, S.R. and R.W. Lowrey. 1973. Cycling of mineral nutrients in pasture ecosystems. In: G.W. Butler and R.W. Bailey (eds.) Chemistry and Biochemistry of Herbage. Vol. 2. Academic Press. London.
51. Blanchet, K., H. Moechnig, and J. DeJong-Hughes. 1999. Grazing Systems Planning Guide. University of Minnesota Extension Service. United States Department of Agriculture. Natural Resources Conservation Service. Accessed at: www.extension.umn.edu/distribution/livestocksystems/DI7606.html.
52. Peterson, P.R. and J.R. Gerrish. 1996. Grazing systems and spatial distribution of nutrients in pastures: Livestock management considerations. In: R.E. Joost and C.A. Roberts (eds.) Nutrient Cycling in Forage Systems. Potash and Phosphate Institute and Foundation of Agronomic Research. Manhattan, KS.
53. West, C.P., A.P. Mallanrino, W.F. Wedin, and D.B. Marx. 1989. Spatial variability of soil chemical properties in grazed pastures. Soil Science Society of America Journal. Vol. 53. No. 3. p. 784-789.
54. Gerrish, J.R., P.R. Peterson, and J.R. Brown. 1994. Planned Grazing Systems for Sustainable Livestock Production. Project 9111031-1.
55. Mueller, J.P. and J.T. Green, Jr. 1995. Controlled grazing. Forage-Livestock Systems. West Virginia University Extension Service. Accessed at: www.caf.wvu.edu/~forage/controlgrazenc/fctrl.htm.
56. Cid, M.S., M.A. Brizuela. 1998. Heterogeneity in tall fescue pastures created and sustained by cattle grazing. Journal of Range Management. Vol. 51. p. 644-649.
57. Forbes, T.D.A. and J. Hodgson. 1985. The reaction of grazing sheep and cattle to the presence of dung from the same or the other species. Grass and Forage Science. Volume 40. p. 177-182.
58. Sharpley, A.N., T. Daniel, T. Sims, J. Lemunyon, R. Stevens, and R. Parry. 1999. Agricultural Phosphorus and Eutrophication. ARS-149. United States Department of Agriculture/Agricultural Research Service. Washington, D.C.
59. Voisin, A. 1960. The different actions of various animal species. In: C.T. Herriott (trans.) Better Grassland Sward. Crosby Lockwood & Son, London.
60. Bishop-Hurley, G.J. 2000. Growth of pasture plants. In: G. J. Bishop-Hurley, S.A. Hamilton, and R. Kallenbach (eds.) Missouri Dairy Grazing Manual. Missouri University Extension. University of Missouri. Columbia, MO.
61. Barnhart, S.K. 1999. How pasture plants grow. Iowa State University. University Extension. Accessed at: www.extension.iastate.edu/Publications/PM1791.pdf.
62. Wienhold, B.J., J.R. Hendrickson, and J.F. Karn. 2001. Pasture management influences on soil properties in the northern great plains. Journal of Soil and Water Conservation. Vol. 56 No. 1. p. 27-31.
63. Turner, C.L., T.R. Seastdt, M.I. Dyer. 1993. Maximization of aboveground grassland production: The role of defoliation frequency, intensity, and history. Ecological Applications. February. p. 175.
64. Sheath. G.W. and D.A. Clark. 1996. Management of grazing systems: Temperate pastures. In: J. Hodgson and A.W. Illus (eds.) The Ecology and Management of Grazing Systems. CAB International. Wallingford, Oxon. UK.
65. Unkovich, M., P. Sandord, J. Pate, and M. Hyder. 1998. Effects of grazing on plant and soil nitrogen relations of pasture-crop rotations. Australian Journal of Agricultural Research. Vol. 49. p.475-85.
66. Aldous, A.E. 1930. Effect of different clipping treatments on the yield and vigor of prairie grass vegetation. Ecology. Vol. 11. p. 752-759.
67. Nie, Z.N., A.D. Mackey, I. Valentine, D.J. Barker, and J. Hodgson. 1997. Influence of pastoral fallow on plant root growth and soil physical and chemical characteristics in a hill pasture. Plant and Soil. Kluwer Academic Publishers. Netherlands. Vol. 197. p. 201-208.
68. Russelle, M.P. 1996. Nitrogen cycling in pasture systems. In: R.E. Joost and C.A. Roberts (eds.) Nutrient Cycling in Forage Systems. Potash and Phosphate Institute and Foundation of Agronomic Research. Manhattan, KS.
69. Henning, J.C. 2001. Managing Pasture Quality. In: T. Terrill and K. Cassida (eds.) American Forage and Grassland Council Proceedings. American Grassland Council. Georgetown, TX.
70. Johnson, T.J. 2001. How Four Legumes Respond to Grazing. Accessed at: www.agron.iastate.edu/moore/434/chapter2.htm.
71. Weaver, J.E. 1926. Root development of field crops. McGraw-Hill Book Company, Inc. New York. Accessed at: www.soilandhealth.org/01aglibrary/010139fieldcroproots/010139ch14.html.
72. Gerrish, J. 2001. Species stability in diverse pasture mixtures. In: T. Terrill and K. Cassida (eds.) American Forage and Grassland Council Proceedings. American Grassland Council. Georgetown, TX.
73. Wedin, D.A. 1995. Species, nitrogen, and grassland dynamics: the constraints of stuff. In: C.G. Jones and J.H. Lawton (ed.) Linking Species & Ecosystems. Chapman & Hall Inc., New York.
74. Killham, K. 1994. Soil Ecology. Cambridge University Press, Cambridge, U.K.
75. Hunt, H.W., D.C. Coleman, E.R. Ingham, R.E. Ingham, E.T. Elliot, J.C. Moore, S.L. Rose, C.P.P. Reid, and C.R. Moreley. 1987. The detrital food web in a shortgrass prairie. Biology and Fertility of Soils. Volume 3. p. 57-68.
76. Holter, P. 1979. Oikos. Volume 32. p. 393-402.
77. Suckling, F.E.T. 1975. New Zealand Journal of Experimental Agriculture. Vol. 3. p. 351-436.
78. Edwards, C. 1999. Soil Biology Primer. United States Department of Agriculture, Natural Resources Conservation Service. Washington, D.C.
79. Soil Quality Institute. 2001. Agricultural management effects on earthworm populations. Soil Quality Technical Note No. 11. United States Department of Agriculture. Natural Resources Conservation Service. Soil Quality Institue. Auburn, AL. Accessed at http://policy.nrcs.usda.gov/scripts/lpsiis.dll/TN/TN_ECS_11_2.htm.
80. United States Department of Agriculture/ Natural Resources Conservation Service. Rangeland Soil Quality—Soil Biota. Soil Quality Information Sheet. Rangeland Sheet 8. Accessed at
http://soils.usda.gov/sqi/files/RSQIS8.pdf.
81. Fincher, G.T. 1981. The potential value of dung beetles in pasture ecosystems. Journal of Georgia Entomological Society. Vol. 16. 1st Supplement. p. 316-333.
82. Richardson, P. Q. and R.H. Richardson. September 1999. Factsheet: Dung beetles (Work for free, love their work). p. 1-3.
83. Knutson, Allen. 2000. Dung beetles—Biological control agents of horn flies. Texas Biological Control News. Texas Agricultural Extension Service, Texas A&M University System. Winter 2000.
84. Yeats, G.W. 1981. Nematode populations in relation to soil environmental factors: a review. Pedobiologia. Vol. 22. p. 312-338.
85. Elliott, E.T., D.C. Coleman, R.E. Ingham, and J.A. Trofymow. 1984. Carbon and energy flow through microflora and microfauna in the soil subsystem of terrestrial ecosystems. In: M. J. Klug and C. A. Reddy (eds.) Proceedings of the Third International Symposium on Microbial Ecology. American Society for Microbiology. Washington, D.C.
86. Soil Quality Institute. Soil Quality—Managing Soil for Today and Tomorrow. Web Page. United States Department of Agriculture, Natural Resources Conservation Service. Accessed at http://soils.usda.gov/sqi/.
87. Garlynd, M.J., A.V. Karakov, D.E. Romig, and R.F. Harris. 1994. Descriptive and analytical characterization of soil quality/health. In: J.W. Doran, D.C. Coleman, D.F. Bezdicek, and B.A. Stewart (eds.) Defining Soil Quality for a Sustainable Environment. Soil Science Society of America, Inc. Madison, WI.
88. Sullivan, P.G. 2001. Assessing the Pasture Soil Resource. ATTRA. Fayetteville. AR.
89. United States Department of Agriculture/Natural Resources Conservation Service. Rangeland Soil Quality—Indicators for Assessment and Monitoring. Soil Quality Information Sheet. Rangeland Sheet 2. Accessed at http://soils.usda.gov/sqi/files/RSQIS2.pdf.
90. Correll, D.L. 1996. Environmental impact of pasture systems on surface water quality. In: R.E. Joost and C.A. Roberts (eds.) Nutrient Cycling in Forage Systems. Potash and Phosphate Institute and Foundation of Agronomic Research, Manhattan, KS.
91. Beegle, D.B., A.N. Sharpley, W.L. Stout, and D.K Bhumbla. 1998. Water quality and land resource protection: Nutrient and manure management in pasture systems. In: C.R. Krueger (ed.) Grazing in the Northeast: Assessing Current Technologies, Research Directions, and Education Needs. Northeast Regional Agricultural Engineering Service. Ithaca, NY.
92. United States Environmental Protection Agency Department of Water. 1999. Laws and Regulations, Policy and Guidance Documents, and Legislation. Web page. Accessed at www.epa.gov/OW/laws.html.
93. Stehman, S.M., C. Rossiter, P. McDonough, and S. Wade. 1996. Potential pathogens in manure. In: J.S. Popow (ed.) Animal Agriculture and the Environment: Nutrients, Pathogens, and Community Relations. Northeast Regional Agricultural Engineering Service. Ithaca, NY.
94. Sharpley, A., T.C. Daniel, J.T. Sims, and D.H. Pote. 1996. Determining environmentally sound soil phosphorus levels. Journal of Soil and Water Conservation. Vol. 51, No. 2. p. 160-166.
95. Heathwaite, L, A. Sharpley, and W. Gburek. 2000. A conceptual approach for integrating phosphorus and nitrogen management at watershed scales. Journal of Environmental Quality. Vol. 29. p. 158-166.
96. Self-Davis, M.L., M.S. Miller, R.L. Raper, and D.W. Reeves. 1996. Pasture soil and vegetation response to renovation tillage. p. 131-136. In: New technology and conservation tillage. Proceedings of the Southern Conservation Tillage Conference for Sustainable Agriculture. Jackson, TN. 23-25 July 1996.
97. Cooke, J.G. 1988. Sources and sinks of nutrients in a New Zealand hill pasture catchment: II. Phosphorus. Hydrology Proceedings. Vol. 2. p. 123-133.
98. Tate, K.W., G.A. Nader, D.J. Lewis, E.R. Atwil, and J.M. Connor. 2000. Evaluation of buffers to improve the quality of runoff from irrigated pastures. Journal of Soil and Water Conservation. Vol. 55, No. 4. p. 473-478.
99. Uusi-Kamppa, J., E. Tutola, H. Hartikainen, and T. Ylaranta. 1997. The interactions of buffer zones and phosphorus runoff. In: N.E. Haycock, T.P. Burt, KW.T. Goulding, and G. Pinay (eds.) Buffer Zones: Their Processes and Potential in Water Protection. Qwest Environmental. Hertfordshire, UK.
100. Mosley, J.C., P.S. Cook, A.J. Griffis, and J. O'Laughlin. 1998. Guidelines for Managing Cattle Grazing in Riparian Areas to Protect Water Quality: Review of Research and Best Management Practices Policy. Accessed at www.uidaho.edu/cfwr/[ag/pag15es.html.
101. Clark, E.A. and R.P. Poincelot. 1996. The Contribution of Managed Grasslands to Sustainable Agriculture in the Great Lakes Basin. The Haworth Press Inc., New York.
102. Lyons, J., B.M. Weigel, L.K. Paine, and D.J. Undersander. 2000. Influence of intensive rotational grazing on bank erosion, fish habitat quality, and fish communities in southwestern Wisconsin trout streams. Journal of Soil and Water Conservation. Vol. 55, No. 3. p. 271-276.
103. Undersander, D. and B. Pillsbury. 1999. Grazing Streamside Pastures. University of Wisconsin Extension. Madison, WI.
104. Clary, W.P. and B.F. Webster. 1989. Managing grazing of riparian areas in the Intermountain Region. Gen. Tech. Rep. INT-263. Ogden, UT: U.S. Department of Agriculture, Forest Service, Intermountain Research Station.
Back to top
Resource List
Agencies and Organizations
American Forage and Grassland Council
Georgetown, TX.
Mission statement: "To promote the use of forages as economically and environmentally sound agriculture through education, communication, and professional development of producers, scientists, educators and commercial representatives and through communication with policy makers and consumers in North America" www.afgc.org.
Grazing Lands Conservation Initiative
A national effort to provide high-quality technical assistance on privately owned grazing lands and increase the awareness of the importance of grazing land resources. www.glci.org/.
Grazing Lands Technology Institute (GLTI)
Provides technical excellence to the Natural Resources Conservation Service (NRCS) and other appropriate customers in the acquisition, development, coordination, and transfer of technology that meets the needs of grazing land resources, landowners and managers, and the public. www.glti.nrcs.usda.gov/, or contact your county or regional NRCS office.
Cooperative State Research, Education, and Extension Service
Educational and technical assistance that links farmers and ranchers with university research expertise. Many county or regional offices address grazing practices. To identify your local office see www.csrees.usda.gov/Extension/index.html.
Natural Resource, Agriculture, and Engineering Service
Ithaca, NY
Coordinates and publishes proceedings from conferences on agricultural and environmental issues. Also publishes technical and practical documents on manure management, composting, and animal housing. www.nraes.org.
Back to top
Publications in Print
General Grazing
Clark, E.A. and R.P. Poincelot. 1996. The Contribution of Managed Grasslands to Sustainable Agriculture in the Great Lakes Basin. The Haworth Press Inc., New York.
An easy-to-read but technically based discussion of the relations between grazing management, forage production, and soil quality.
Emmick, D.L. and D.G. Fox. 1993. Prescribed Grazing Management to Improve Pasture Productivity in New York. United States Department of Agriculture Soil Conservation Service and Cornell University. www.css.cornell.edu/forage/pasture/.
Gerrish, J. and C. Roberts (eds.) 1996 Missouri Grazing Manual. University of Missouri. Columbia, MO.
Short papers addressing forage production and nutrient management in pastures.
Pearson, C.J. and R.L. Ison. 1987. Agronomy of Grassland Systems. Cambridge University Press. Cambridge.
Technical discussion of forage biology and production, nutrient availability, and animal nutrition on forages.
Stockman Grass Farmer Magazine
The nation's leading publication on grass-based livestock systems. Order information http://stockmangrassfarmer.com/.
Terrill, T. and K. Cassida. 2001. American Forage and Grassland Council Proceedings. American Forage and Grassland Council. Georgetown, TX.
Unsander, D., B. Albert, P. Porter, A. Crossley, and N. Martin. No date. Pastures for Profit. A Guide to Rotational Grazing. University of Wisconsin Extension. Madison, WI. Available at http://www.uwrf.edu/grazing/.
Soil Quality and Soil Conservation
Magdoff, F. and H. van Es. 2000. Building Soils for Better Crops, Second Edition. Sustainable Agriculture Network. Handbook Series Book 4. Beltsville, MD.
Easy-to-read descriptions of concepts regarding soil quality and agricultural management practices to enhance soil quality. Although many of the practical management descriptions are oriented more towards field crops than to grazing systems, the concepts of soil quality protection and management are the same.
Edwards, C. 1999. Soil Biology Primer. United States Department of Agriculture, Natural Resources Conservation Service. Washington, D.C. For an online version or order information: http://soils.usda.gov/sqi/concepts/soil_biology/index.html
A well-illustrated overview of soil organisms and their impact on soil quality.
Cavigelli, M.A., S.R. Deming, L.K. Probyn, and R.R. Harwood (eds). 1998. Michigan Field Crop Ecology: Managing biological processes for productivity and environmental quality. MSU Extension Bulletin E-2646. Michigan State University Extension Service, East Lansing, MI.
A well-illustrated overview of nutrient cycles in agricultural systems, the organisms that affect these systems, and the impact of environmental conditions and management practices on the activities of these organisms.
Journal of Soil and Water Conservation. Technical articles on soil conservation research and practices. Many articles pertain to grazing systems. Order information: www.swcs.org/en/journal_of_soil_and_water_conservation/
Whitehead, D.C. 2000. Nutrient Elements in Grassland Soil-Plant-Animal Relationships. CAB International Publishing. Wallingford, Oxon, UK.
An excellent technical resource on nutrient cycle components and interactions in grazing systems.
Undersander, D. and B. Pillsbury. 1999. Grazing Streamside Pastures. University of Wisconsin Extension. Madison, WI.
An easy-to-read overview of the potential risks and benefits associated with riparian grazing practices.
Water Quality Protection
Sharpley, A.N., T. Daniel, T. Sims, J. Lemunyon, R. Stevens, and R. Parry. 1999. Agricultural Phosphorus and Eutrophication. ARS-149. United States Department of Agriculture / Agricultural Research Service. Washington, D.C. Order information: www.soil.ncsu.edu/sera17/publicat.htm.
An illustrated, easy-to-read discussion describing the impact of environmental conditions and land management practices on the risk for phosphorus runoff from agricultural lands.
Daniels, M., T. Daniel, D. Carman, R. Morgan, J. Langston, and K. VanDevender. 1998. Soil Phosphorus Levels: Concerns and Recommendations. University of Arkansas. Division of Agriculture. Cooperative Extension Service. Accessed at: www.soil.ncsu.edu/sera17/publicat.htm.
NRAES. 2000. Managing Nutrients and Pathogens from Animal Agriculture. Natural Resource, Agriculture, and Engineering Service. Ithaca, NY.
Proceedings from a conference that included research reports, field experience, and policy discussions. Available from www.nraes.org.
Back to top
Web Resources
Rotational Grazing—University Programs
Center for Grassland Studies—University of Nebraska-Lincoln
www.grassland.unl.edu/index.htm
Purdue Pasture Management Page—Purdue University Cooperative Extension
www.agry.purdue.edu/ext/forages/rotational/
Texas Cooperative Extension Bookstore
http://texaserc.tamu.edu/catalog/query.cgi?id=433
Controlled Grazing of Virginia's Pastures—Virginia Cooperative Extension
www.ext.vt.edu/pubs/livestock/418-012/418-012.html
Grazing Dairy Systems at the Center for Integrated Agricultural Systems—University of Wisconsin—Madison
www.cias.wisc.edu/production.php#grazing
Pasture Management & Grazing—University of Wisconsin Extension
www.uwrf.edu/grazing/
Grasslands Watershed Management—Clemson University
http://grasslands.clemson.edu/
Focuses on the role pasture and forage crop production can play in helping insure a clean, safe water supply.
Rotational Grazing—Organizations and Agencies
Grazing Lands Conservation Initiative
www.glci.org/
A national effort to provide high-quality technical assistance on privately owned grazing lands and increase the awareness of the importance of grazing land resourses.
Grazing Lands Technology Institute—Grazing information from the Natural Resources Conservation Service
www.glti.nrcs.usda.gov/
Stockman Grass Farmer Magazine—The nation's leading publication on grass-based livestock systems
http://stockmangrassfarmer.com/
American Farmland Trust information site on grass-based farming systems
http://grassfarmer.com/
Sustainable Farming Connection's Grazing Menu—good links to grazing sites
www.ibiblio.org/farming-connection/grazing/home.htm
Why Grassfed Is Best—Jo Robinson explores the many benefits of grassfed meat, eggs, and dairy products
www.eatwild.com/
Archived listings from Graze-L, an international forum for the discussion of rotational grazing and seasonal dairying
http://graze-l.witt.ac.nz/pipermail/graze-l/
Soil Quality
Soil Quality Institute. United States Department of Agriculture. Natural Resources Conservation Service
http://soils.usda.gov/sqi/
Soil quality information sheets, soil quality indicators, and soil quality assessment methods.
Rangeland Soil Quality. Soil Quality Institute. United States Department of Agriculture Natural Resources Conservation Service
http://soils.usda.gov/sqi/
Information sheets on rangeland soil quality.
Soil Biological Communities. United States Department of Agriculture Bureau of Land Management. Information sheets
www.blm.gov/nstc/soil/index.html
Ingham, E. 1996. The soil foodweb: Its importance in ecosystem health
http://rain.org:80/~sals/ingham.html
The Soil Foodweb Incorporated
www.soilfoodweb.com/
Soil microbiology, soil ecology information and laboratory analyses of soil biology.
SoilFacts. Soil-science-related publications from North Carolina State University
www.soil.ncsu.edu/about/publications/index.php
Includes: Poultry Manure as a Fertilizer Source, Good Soil Management Helps Protect Groundwater, Nitrogen Management and Water Quality, and Soils and Water Quality.
United States Department of Agriculture—Agricultural Research Service
www.ars.usda.gov/research/programs.htm
Research programs addressing soil and water quality, rangeland, pastures, and forests, and integrated agricultural systems.
Water Quality
Pellant, M., P. Shaver, D.A. Pyke, and J.E. Herrick. 2000. Interpreting Indicators of Rangeland Health. Version 3. Technical Reference 1734-6. National Sciene and Technology Center Information and Communications Group. Denver, CO.
www.glti.nrcs.usda.gov/technical/publications/index.html#range-health-indicate
SERA-17. Minimizing Phosphorus Losses from Agriculture.
www.soil.ncsu.edu/sera17/publicat.htm
National, multi-agency information on phosphorus fate and transport, including the development of the phosphorus index.
United States Environmental Protection Agency Department of Water. 1999. Laws and Regulations, Policy and Guidance Documents, and Legislation.
www.epa.gov/OW/laws.html
Concentrated Animal Feeding Operations (CAFOs) Effluent Guidelines for larger scale farms.
www.epa.gov/ost/guide/cafo/index.html
Focuses on confinement systems but many of the nutrient management planning guidelines may also be appropriate for grazing systems.
Animal Feeding Operations. US EPA Office of Water.
http://cfpub.epa.gov/npdes/home.cfm?program_id=7
EPA's National Agriculture Compliance Assistance Center.
www.epa.gov/agriculture/
Information on environmental laws affecting agricultural operations.
Riparian Grazing
Riparian Grazing Project—University of California Cooperative Extension
http://wsare.usu.edu/pro/pr2003/SW01-044.pdf
Effects of Cattle Grazing in Riparian Areas of the Southwestern United States
www.earlham.edu/~biol/desert/riparian.htm
Managed Grazing and Stream Ecosystems. Laura Paine and John Lyons
www.uwrf.edu/grazing/
Driscoll, M. and B. Vondracek. 2001. An Annotated Bibliography of Riparian Grazing Publications. The Land Stewardship Project
www.landstewardshipproject.org/resources-main.html
By Barbara Bellows
NCAT Agriculture Specialist
Copyright © 2001 National Center for Appropriate Technology
IP136
Slot 49
Back to top |