posted by Dr. Amber Jenkins
17:00 PDT

They say a picture says a thousand words. This week we published our 100th image in State of Flux, our gallery showing images of change around our planet. So hopefully by now you’re in awe of our home planet and the ways in which it is constantly changing, and aware of the impact us humans can have.
Each week for the past couple of years, we’ve published new images of different locations on planet Earth, showing change over time periods ranging from centuries to days. The pictures have been taken from space, by NASA’s Eyes on the Earth (its fleet of satellites whizzing above our heads), and from the ground, by real-life people. Some of the changes seen are related to, or exacerbated by, climate change, and some are not. Some document the effects of urbanization and man’s impact on the land, while others the ravage of disasters such as fires and floods.
Seeing our planet from space gives us a global view that we can’t get elsewhere. Through those eyes, we’ve witnessed damage caused by the recent tsunami in Japan, glacier melt in the Himalayas, the greening of China, the growth of Las Vegas and a century of global warming. We’ve looked at the march of deforestation in Bolivia, the rumblings of the (unpronounceable) Icelandic volcano Eyjafjallajökull, and the damming of the River Nile. Take a look below at some of our favorites. Sign up to our monthly newsletter or subscribe to our Facebook page if you want to keep up to date with our latest images. We’ll be launching a brand spanking new version of the gallery soon!






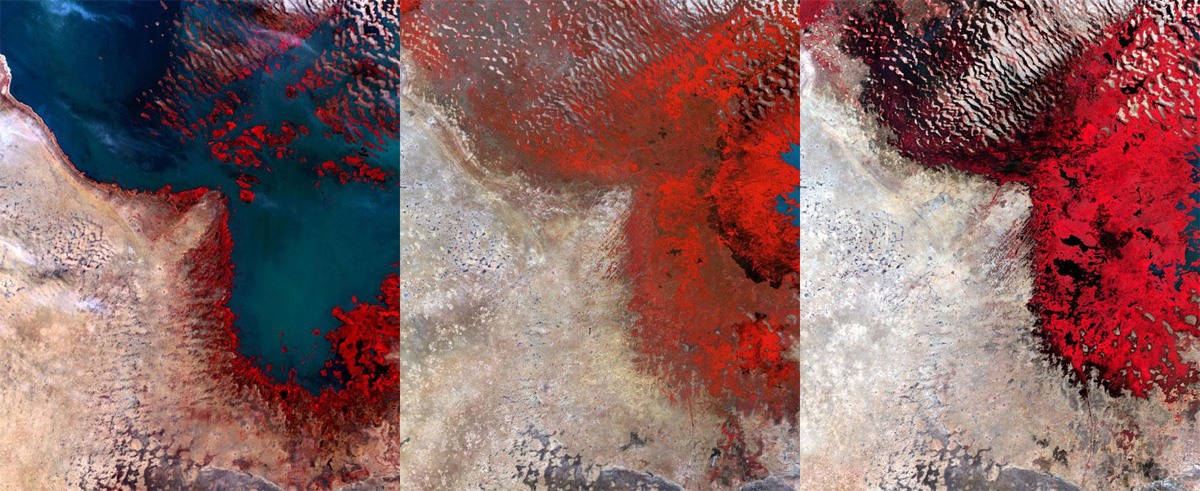


