How Lasers Work
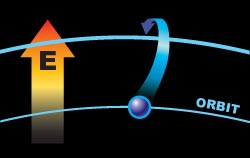

A laser can be as small as a microscopic computer chip or as immense as the National Ignition Facility (NIF), which is three football fields wide. Clearly size has nothing to do with what makes a laser. "Laser" is an acronym for light amplification by stimulated emission of radiation. If the electrons in special glasses, crystals, or gases are energized, they will emit light photons in response to a weak laser pulse. The photons will all be at the same wavelength and will also be "coherent," meaning the light wave's crests and troughs are all in lockstep. In contrast, ordinary visible light comes in multiple wavelengths and is not coherent.
In NIF, as in most large lasers, intense flashes of white light from giant flashlamps "pump" the atomic electrons in large slabs of laser glass to a higher or more "excited" energy state. But this excited state is transitory, lasting only about one-millionth of a second. A small pulse of laser light "tuned" to the excited electrons' energy is directed through the glass slabs. This laser pulse stimulates the electrons to drop to their lower energy states, or "ground" states, and emit a laser photon of exactly the same wavelength.
A system of mirrors at both ends of the laser glass amplifier causes the photons to travel back and forth through the glass, stimulating more electrons to drop to their lower energy states and emit laser photons. This process results in "amplification," or the production of huge numbers of photons of the same wavelength and direction. In NIF, amplification produces trillions upon trillions of photons—1023 photons to be precise (see Amplifiers). As the photons encounter each other inside the laser amplifier, they vibrate coherently with the same frequency and direction. In essence, the photons "clone" themselves, creating a perfect copy of the first photon. Coherence causes the light to shine in a beam that is extremely bright and straight, familiar to anyone who has used a laser pointer.
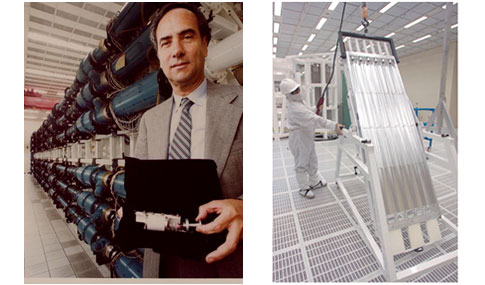
The initial weak pulse has by now been amplified and increased enormously in energy. In small lasers, a partially reflecting mirror at one end of the lasing medium is designed to allow some of the amplified light—now laser light—to pass through (see "The First Ruby Laser" below). In NIF, a special optical switch traps the low-energy laser pulse in the main amplifier for four passes back and forth through the laser glass slabs. Only then are the laser beams switched out of the main amplifier to continue their journey to the target chamber. This system of glass, mirrors, and other special optics is known as a laser amplifier cavity. Precise design of the amplifier components allows scientists to control how much energy the laser beam will produce. In NIF, a series of amplifiers increases the energy of the initial weak pulse of light by more than a quadrillion times to create NIF's 192 highly energetic, tightly focused laser beams.
The First Ruby Laser
The ruby laser was the first laser invented in 1960. Ruby is an aluminum oxide crystal in which some of the aluminum atoms have been replaced with chromium atoms. Chromium gives ruby its characteristic red color and is responsible for the lasing behavior of the crystal. Chromium atoms absorb green and blue light and emit or reflect only red light.
For a ruby laser, a crystal of ruby is formed into a cylinder. A fully reflecting mirror is placed on one end and a partially reflecting mirror on the other. A high-intensity lamp is spiraled around the ruby cylinder to provide a flash of white light that triggers the laser action. The green and blue wavelengths in the flash excite electrons in the chromium atoms to a higher energy level. Upon returning to their normal state, the electrons emit their characteristic ruby-red light. The mirrors reflect some of this light back and forth inside the ruby crystal, stimulating other excited chromium atoms to produce more red light, until the light pulse builds up to high power and drains the energy stored in the crystal.
1. High-voltage electricity causes the quartz flash tube to emit an intense burst of light, exciting some of the atoms in the ruby crystal to higher energy levels.
2. At a specific energy level, some atoms emit particles of light called photons. At first the photons are emitted in all directions. Photons from one atom stimulate emission of photons from other atoms and the light intensity is rapidly amplified.
3. Mirrors at each end reflect the photons back and forth, continuing this process of stimulated emission and amplification.
4. The photons leave through the partially silvered mirror at one end. This is laser light.
The laser flash that escapes through the partially reflecting mirror lasts for only about 300 millionths of a second, but is very intense. Early lasers could produce peak powers of some 10,000 watts. Modern lasers can produce pulses that are billions of times more powerful.
The 1,000-watt light used in modern lighthouses can be seen from 20 miles away. But beyond that distance, the light beam has spread out so much that it is difficult to see. A laser beam, however, will run straight and true for very long distances. A laser beam has been bounced off the moon to accurately measure the distance of more than 250,000 miles! The beam stays roughly the same size as it travels this vast distance. Coherence means that laser light can be focused with great precision.
Many different materials can be used as lasers. Some, like the ruby laser, emit short pulses of laser light. Others, like helium–neon gas lasers or liquid dye lasers, emit a continuous beam of light. The NIF lasers, like the ruby laser, are solid-state, pulsed lasers.