Target Fabrication
Radiograph of a high-density carbon capsule with a smooth, frozen layer of D-T inside.All of the experiments at the National Ignition Facility require a target precisely located in the center of the NIF target chamber. Just a few millimeters long, NIF targets are complicated, precision assemblies, often requiring novel material structures. Creating these targets is a complex interplay among target designers, materials scientists and precision engineers. The target designers know what each experiment must achieve and define the target specifications, which include size, shape, density, concentricity and surface smoothness. Materials scientists work to create the essential raw materials, while fabrication engineers determine whether the materials – sometimes entirely new and unique – can be machined and assembled. The NIC team is perfecting ICF experiment target fabrication and materials, as well as advancing laser driver performance, target design and the performance of experimental diagnostics.
Materials scientists and engineers from Livermore's Nanoscale Synthesis and Characterization Laboratory also are performing research in target fabrication as part of a Laboratory Directed Research and Development Strategic Initiative. This project team is working on the synthesis of new materials and inventing new fabrication techniques in support of future fusion experiments beyond NIC, stockpile stewardship and basic science. Some of the new nanoscale materials include high-density carbon, copper and gold foams and graded-density foams. Each serves different functions in various target components (see Target Physics).
Manufacturing requirements for all NIF targets are extremely rigid. Components have to be machined to an accuracy of within one micrometer, or one millionth of a meter. Some joints can be no larger than 100 nanometers, which is just 1,1000th of a human hair's width. Precise microassembly of the targets has a margin for error of less than three microns. The extreme temperatures and pressures the targets will encounter during experiments make the results highly susceptible to any imperfections in fabrication. New tools to image and characterize materials provide insight into development progress and necessary changes in material preparation and fabrication. Continual improvements are also based on simulations and experiments with the Janus laser at LLNL, the OMEGA laser at the University of Rochester, and other facilities worldwide.
The Demands of ICF

To achieve ignition, the target design contains four essential components: deuterium and tritium (D-T) fuel, a capsule with fill tube, a hohlraum and thermal control hardware.
NIF's powerful laser beams impinge on the inside of a hohlraum (German for "hollow space"), where the laser energy is converted to X-ray energy. These X-rays bathe the fuel capsule and rapidly ablate, or burn away, the capsule's outer layer. The principle of conservation of momentum (every action requires an equal and opposite reaction) forces the remaining material to implode or compress. Compression of the D-T fuel – which has been formed in an ice layer inside the capsule – to extraordinarily high temperature, pressure and density ignites a burning hydrogen plasma. To assure the symmetry of the implosion, the capsule must be placed within eight microns of the center of the hohlraum, which is itself just one centimeter across.
The Fuel
Laboratory scientists have pioneered procedures, including a seeded growth technique, to precisely form the frozen layer of D-T fuel inside the fuel capsule. The temperature of the D-T ice is 1.5 degrees below the "triple point" of the hydrogen isotope mixture – the temperature at which all three phases of the substance can coexist in equilibrium – and controlled with a temperature stability of one thousandth of a kelvin. Heat released by the beta decay of the tritium helps to smooth the layer by selectively heating thicker regions and evaporating hydrogen from them.
The roughness of the D-T ice layer is determined by a combination of seeding (initial layer formation) and the cooling protocol used to reduce the temperature from the triple point. Within 36 hours of introducing the D-T gas inside the capsule, the target must be shot due to the adverse effects of a buildup of helium that is the product of the decay of the tritium.
The Capsule
The capsule or ablator is made from light or low-atomic-number elements that perform well as "rocket fuels" when ablated by the X-rays in the hohlraum. One design uses beryllium for the capsule and includes a radially tailored copper dopant to fine-tune its absorption of the X-rays as well as a smooth solid layer of D-T fuel on its inner surface. To make ignition work, the beryllium capsule needs to have a precise spherical shape. Its surfaces must be smooth to the nanometer (billionth of a meter) level with carefully controlled copper-doped layers of uniform thickness and controlled opacity. Filling the capsule with D-T gas requires a small fill hole less than five microns across drilled through 150 microns of beryllium. Research to achieve these specifications is being conducted at General Atomics and LLNL.
Production of the capsule starts with a very smooth, very spherical plastic mandrel, the form on which the beryllium is deposited. Beryllium is "sputtered," or deposited, onto the mandrel as the mandrel is rolled or rotated. Very carefully, a 150-micron-thick layer of beryllium is built up on the mandrel's surface. Following deposition, the capsules are polished and the five-micron fill hole is laser-drilled. Because the mandrel is plastic, it can be removed through the drilled hole by carefully heating the shell in an oxygen-rich atmosphere to "burn" or pyrolyze the plastic, resulting in gases which can escape through the small fill hole. A precisely drawn and finished tube of glass or polyimide is used to make the ten-micron fill tube which is attached to the capsule so it can be filled with D-T gas.
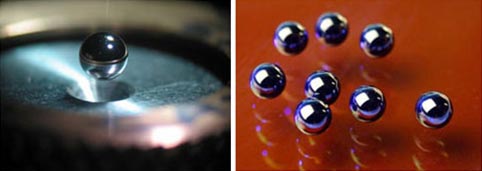
Right: 2-mm polished high-density carbon ablator capsules with the silicon mandrel inside.
A possible alternative to the beryllium capsule is one made of high-density carbon. Like beryllium, carbon is a low-atomic-number material, and its high density makes it an attractive material for an ICF capsule. Laboratory physical chemists have teamed up with researchers at the Fraunhofer Institute for Applied Solid State Physics of Freiburg, Germany, on this technology for fuel capsules. They have developed techniques for depositing high-density carbon films on two-millimeter silicon mandrels for polishing the spheres and for removing the silicon.
The Hohlraum
The capsule is fitted inside a hohlraum cylinder measuring just nine millimeters high by five millimeters in diameter. In radiation thermodynamics, a hohlraum is defined as "a cavity whose walls are in radiative equilibrium with the radiant energy within the cavity." The hohlraum is made from a high-atomic-number material (an element with a high electron density) such as gold and uranium.
Researchers at General Atomics have developed hohlraum fabrication techniques that couple as much laser energy to the capsule as possible. The ignition hohlraum is a seven-micron-thick gold- and uranium-layered cylinder encapsulated in gold to prevent oxidation and provide structural rigidity. The layers are sputter-deposited on a precision-machined mandrel, which is leached away after deposition and machining are complete.
The Thermal Control Package
The Thermal-Mechanical package integrated with the hohlraum and capsule assembly.The geometric shape of the D-T ice is determined by the details of the heat transfer between the hohlraum and the capsule. Precise control of this heat transfer is needed to form a spherical ice layer in a cylindrical can. This is achieved through the integration of a thermal-mechanical package (TMP) with the hohlraum and capsule assembly. The TMP consists of two precision-fabricated aluminum shells joined by a precision-fabricated band with cutouts to accommodate various diagnostics requirements. The design is modular and will be used throughout the ignition campaign.
Two silicon "cooling arms" attached to either end of the TMP assembly are used to conduct heat away from the hohlraum to maintain the required temperature. These are lithographically etched to create a precise heat transfer path that ensures temperature uniformity in the target. Heaters located on the TMP shells are then used to produce a nearly spherical isotherm, or surface of equal temperature, around the capsule.
The Assembly
A prototype ignition target.To meet the high target production demands of NIC, the target fabrication team has had to focus on designing for manufacturability and reproducibility. This is achieved during component fabrication and subassembly at General Atomics and in final assembly at LLNL (View video). Rigorous measurement and precision components couple to make these assemblies reproducible, so that each experiment leading to ignition has an identical design basis.
Once assembly is complete, the target will be integrated with the cryogenic target positioning system (CTS) in NIF. This system will form and characterize the D-T layer and will place the target at target chamber center for the shot. Target position will be maintained to within two microns, and temperature will be held in the range of 18 to 20 kelvins (-427 to -424 degrees Fahrenheit) with a stability of one thousandth of a kelvin.
Targets for Science
The science and technology capabilities that have been and are being established for fabrication of targets will not only support the high energy density science mission of LLNL and NNSA, but also many other science missions for the nuclear weapons complex and the nation. The ability to create novel materials with precise fabrication methods and characterization techniques is an enabling element of NNSA science and high energy density science campaigns.
More Information
"Meeting the Target Challenge," Science & Technology Review, July/August 2007
"Fusion Targets on the Double," Science & Technology Review, September 2006
"Lightweight Target Generates Bright, Energetic X-Rays," Science & Technology Review, October 2005