
Remarks

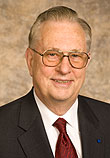 |
Dr. Arden L. Bement, Jr.
Director
National Science Foundation
Biography
Climate Change: Challenges and Priorities for Basic Research
Tokyo, Japan
May 20, 2008
See also slide presentation.
|
[Slide 1: Title slide]
(Use "back" to return to the text.)
Greetings to everyone. I am delighted to be here in Japan once again, and to share with you some thoughts on a topic of great importance to all of us.
As Director of the U.S. National Science Foundation, I am in the new knowledge business. Our mission is to support science and engineering research at the frontiers of discovery. This is the unexplored territory where new ideas are born, nurtured and eventually bear fruit in applications that improve our prosperity and well being.
Among the many competing research challenges of our times, some stand out because they are so vital for social well-being that we have an obligation to attack them head-on.
Today, I will focus my comments on one of the most urgent and pervasive challenges for science and technology in the 21st Century: global climate change.
[Slide #2: Japanese Cherry Blossoms]
(Use "back" to return to the text.)
The image of cherry blossoms has deep cultural resonance here in Japan. But it has also become a symbol of environmental change.
As you know, the dates of cherry blossom festivals have emerged as one of the most important sources of information on how climate change is affecting plants. The official advent of sakura has moved up by 4.2 days since bloom times were first recorded almost a millennium ago.
Observations such as these help us to tease out the complex patterns of long-term climate change from the vagaries of ever-changing weather. Scientists have been tackling this difficult problem for more than fifty years. We are in a much better position today to understand the magnitude and complexity of climate change.
I'll speak today about some of those challenges and how research can help us resolve them.
[Slide #3: Chart From IPCC: Global Average Warming and Sea Level, Northern Hemisphere Snow]
(Use "back" to return to the text.)
The physics of climate change tells us that temperatures and sea level are likely to continue rising for decades to come, even under the most stringent mitigation regimes. We can certainly lessen future impacts on people and the environment, but we cannot entirely prevent them. Adapting to change will require new technologies, informed by robust science.
The recently released IPCC assessment is an encouraging account of rapid advances in our understanding of many aspects of climate change and its impacts. It also points to areas of research where we need to make rapid progress in the future
[Slide #4: Rapid Changes in Arctic Systems]
(Use "back" to return to the text.)
Let me begin with a view from the "top" of the world. There is evidence that the Arctic is experiencing greater environmental change than any other region of the planet. The scope and scale of these changes are unprecedented in recorded history.
Moreover, the complex and dynamic Arctic system has demonstrated the capacity for rapid, amplified, and unpredictable change, with both local and global implications.
[Slide #5: Shrinking Greenland Ice Sheet]
(Use "back" to return to the text.)
Glaciers in the Arctic are retreating and thinning, a phenomenon that is not confined to the Arctic, but is global in scope.
The melting of the Greenland ice sheet raises concerns about rising sea level. There is enough frozen water in Greenland to raise global sea levels seven meters.1
[Slide #6: Arctic Sea Ice Minimums]
(Use "back" to return to the text.)
Sea ice cover in the Arctic is also retreating and thinning, a visible indicator of warming. We don't as yet understand the complex and multiple feedback mechanisms involved that may influence future climate or the global variability in climate change.
[Slide #7: Meridional Overturning Circulation]
(Use "back" to return to the text.)
And as the ice sheet melts, along with other northern glaciers and sea ice, fresher and less dense water flows into the North Atlantic Ocean, creating seawater of lower density. This water, diminished in density, may be weakening ocean circulation.
[Slide #8: Melting Permafrost]
(Use "back" to return to the text.)
On land, permafrost is melting in some areas, raising questions about possible impacts on seasonal lakes and the wildlife and humans that depend upon them.
[Slide #9: Ecosystem Changes]
(Use "back" to return to the text.)
Arctic ecosystems show changes, including earlier bloom-time for some Arctic flora, and variability in migration for fauna due to changes in relative floral abundance.
[Slide #10: Changing Climate, Changing Lives]
(Use "back" to return to the text.)
Arctic inhabitants are already experiencing significant change in their daily lives. Increasing temperatures and rising sea levels are likely to have similar, far-reaching effects on ecosystems and people in every corner of the world.
[Slide #11: Wilkins Ice Shelf Collapse]
(Use "back" to return to the text.)
At the "other end" of the Earth, in Antarctica, we can observe equally troubling signs of change. Scientists recently witnessed the collapse of the Wilkins ice shelf.
The IPCC report singles out a major source of uncertainty in forecasts of the future effects of climate change: namely, how will the earth’s major ice sheets contribute to sea level rise?
[Slide #12: Collapse of Larsen B Ice Shelf]
(Use "back" to return to the text.)
Researchers are now investigating abrupt environmental change in the Larsen Ice Shelf. Before its collapse in 2002, this ice shelf buttressed the glaciers behind it, slowing their inexorable movement to the sea.
After the collapse, the rate of this flow increased eight-fold. Researchers are investigating how such abrupt change affects interlinked processes in the oceans, the ice, and the ecosystem that has existed under the ice shelf, undisturbed, for thousands of years. Teams from the U.S., UK, Argentina, Belgium, Germany and Spain are partners in this activity.
[Slide #13: Fate of Polar Ice Sheets?]
(Use "back" to return to the text.)
Another team of researchers is investigating similar processes in the Amundsen Sea Embayment region of West Antarctica where ice shelves remain intact. Unlike the much smaller glaciers and ice caps in the northern Antarctic Peninsula, the massive ice sheets2 that flow into the Amundsen Sea contain enough water to raise sea level by five meters.
A significant objective of the research is to improve our ability to predict what will likely be the fate of this enormous system--and its impact on sea level--as temperatures warm.
[Slide #14: Changing Climate]
(Use "back" to return to the text.)
What we observe at the Poles is a harbinger for what may soon be in store for the rest of the planet. The impacts of climate change are amplified and swifter in polar and Alpine regions, but in every region of the world, observations of climate change effects are mounting.
[Slide #15: Population Concentration on Coasts]
(Use "back" to return to the text.)
This view of the world only hints at the concentration of population in coastal regions. Ten percent of the world’s population lives in coastal areas that are less than 10 meters [33 feet] above sea level: that's 600 million people. About 360 million of these live in urban areas, which are particularly vulnerable to increases in coastal storms and rising sea levels
[Slide #16: Global Average Temperature]
(Use "back" to return to the text.)
The IPCC projections of what may happen--if we do nothing--are a formidable call to action. This image shows the extent of global warming if we continue on the current trajectory.
The present impacts of global climate change, as well as others we cannot yet foresee, are likely to exacerbate the problems already faced by millions of the planet's inhabitants.
As temperatures and sea levels continue to rise, we can anticipate a growing list of impacts on people and the environment.
[Slide #17: Eroding Coastlines]
(Use "back" to return to the text.)
From coastal erosion, to ...
[Slide #18: Droughts]
(Use "back" to return to the text.)
Increased drought and famine ...
[Slide #19: Floods]
(Use "back" to return to the text.)
Flooding ...
[Slide #20: Forest Fires]
(Use "back" to return to the text.)
Forest fires ...
[Slide #21: Loss of Biodiversity]
(Use "back" to return to the text.)
Loss of biodiversity ...
[Slide #22: Severe Weather Events]
(Use "back" to return to the text.)
And more frequent severe weather events.
Clearly, we want to minimize the potential impacts on people and the environment. And we want to design policies that achieve this efficiently and effectively.
[Slide #23: Moving Forward]
(Use "back" to return to the text.)
We do not yet fully understand the changes occurring at the Poles and around the planet, or even why the changes are different between the Poles and among different regions of the Poles. New tools are available to help make the needed observations and synthesis. They range from satellites to ships to sensors, and from genomics to nanotechnology, information technology, and advances in remote and robotic technologies.
[Slide #24: POLENet Installations]
(Use "back" to return to the text.)
The Polar Earth Observing Network, a consortium involving participants from 28 nations--including Japan and the U.S.--is expanding the coverage of many different kinds of geophysical data across the Polar Regions.
[Slide #25: Arctic Observing Network]
(Use "back" to return to the text.)
e Arctic Observing Network, or AON, significantly advances our observational capability in the Arctic. AON consists of atmospheric, land and ocean-based environmental monitoring capabilities that allows us to integrate data on a range of environmental changes.
Observatories are essential for advancing climate change science across the board, not merely to address polar science. There is need for a global system of ocean observatories to elucidate pressing issues, including changes in ocean circulation, ocean acidification, and exploration of complex biogeochemical ocean processes.
[Slide #26: Two Decades of Ocean Time Series Research Reveals Changes in the Surface Ocean Environment]
(Use "back" to return to the text.)
The rising concentration of carbon dioxide in the oceans has been called "the other carbon problem." Researchers believe that seawater chemistry will change in coming decades and centuries in ways that will dramatically alter marine life. Marine organisms--including corals--that secrete skeletal structures and help support ocean biodiversity, may be threatened by an ocean turned more acidic as a result of carbon dioxide absorbed by the seas.
We are only beginning to understand these complex interactions between large-scale chemistry changes and marine ecology.
[Slide #27: Deploying Ocean Buoy in North Pacific]
(Use "back" to return to the text.)
The first buoy designed exclusively to monitor increasing acidity in the ocean was launched last year in the Gulf of Alaska, a region likely to be one of the first to feel the impacts of this ocean change.
The broader goal of this research is to examine how ocean circulation and ecosystems interact to determine how much carbon dioxide the North Pacific Ocean absorbs each year.
[Slide #28: The Atmosphere-Ocean Balance]
(Use "back" to return to the text.)
Of the approximately 8 billion tons of carbon emitted each year, about 40 percent accumulates in the atmosphere and about 30 percent is absorbed by the oceans. Scientists believe that terrestrial ecosystems, especially trees, are taking up the remainder. How much carbon is sequestered below the ground surface is still entirely unknown.
Furthermore, we do not yet have a detailed account of the global distribution of carbon sinks, or the limits of their capacity to absorb CO2. Without that critical information, it will be difficult to judge the relative efficiency and effectiveness of mitigation and adaptation proposals.
[Slide #29: Carbon Cycling in the Oceans]
(Use "back" to return to the text.)
One recent study indicates that a fraction of the carbon in the ocean surface never reaches the deep ocean, where it can be stored and prevented from re-entering the atmosphere as a greenhouse gas. Instead of sinking, carbon dioxide is often consumed by animals and bacteria and recycled in the "twilight zone," a dimly lit area 100 to 1,000 meters below the surface.
Researchers found that only 20 percent of the total carbon in the ocean surface made it through the twilight zone off Hawaii, while 50 percent did in the northwest Pacific near Japan.
I offer these two examples as illustrations of how new knowledge continually refines our understanding--and can sometimes alter our assumptions, with implications for mitigation and adaptation strategies.
[Slide #30: Carbon Sequestration in Forests]
(Use "back" to return to the text.)
Another study challenges conventional assumptions by concluding that intact tropical forests are removing an unexpectedly high proportion of carbon dioxide from the atmosphere, thereby partially offsetting carbon entering the air through industrial emissions and deforestation.
That means that forests in the United States and other northern mid- and upper-latitude regions are playing a smaller role in offsetting global warming than previously thought.
Filling gaps in our understanding of carbon sequestration and fully delineating the carbon cycle is one of the great challenges for current climate science.
[Slide #31: Climate Models]
(Use "back" to return to the text.)
Today's climate research relies on highly sophisticated computer models to explore the complexities and project the potential impacts of increasing temperatures. Without these models, it would be nearly impossible to anticipate the extent and severity of potential changes that may lie ahead. Model forecasts give us a window on the future.
The simulations made possible by high-performance computing have provided some of the most valuable insights in climate science. As you know, Japan's Earth Simulator plays a prominent role here. We need many more such resources as we continue to increase the resolution of our climate models.
[Slide #32: Earth Systems Grid]
(Use "back" to return to the text.)
We will also need advanced cyberinfrastructure to handle the floods of data from vastly improved observing systems and model simulations. The Earth Systems Grid is one example of what can be accomplished using advanced cyberinfrasructure tools.
This Grid is a virtual collaborative environment that draws on cyberinfrastructure resources at the National Center for Atmospheric Research, and four Department of Energy national laboratories.
The ESG now includes the past 6 years of joint NSF-funded NCAR and Department of Energy climate modeling experiments, amounting to around 198 terabytes of information. There are some 8000 registered users.
A special IPCC portal contains 35 terabytes of model data from thirteen countries, generated by a modeling campaign organized by IPCC. Over 400 scientific articles have been published using this database.
Understanding how these changes mesh within a complex system is vital to inform decisions that will shape our response as a global community. And new knowledge, together with the new technology it spawns, is a prerequisite for crafting those solutions intelligently.
[Slide #33: Confronting the Impacts]
(Use "back" to return to the text.)
It is not only possible to make progress, it is necessary that we do so. Nobel Prize-winner Paul Crutzen has coined the phrase "Anthropocene Age"3 to describe the present day as the first geological era in which human actions have resulted in impacts on our environment that are planetary in scale.
Through advances in science and technology, we have come to understand the need to craft a future in which our actions as a global community are sustainable.
One aspect of sustainability is that we should meet the needs of our own times without compromising the ability of future generations to meet theirs.4
[Slide #34: Growth in World Population, 1950-2050]
(Use "back" to return to the text.)
Most projections see world population growing from the current six billion to at least nine billion by about 2050. Today, many of those six billion are without adequate nutrition, plentiful and safe water, and reliable energy supplies.
[Slide #35: Energy]
(Use "back" to return to the text.)
I have not yet addressed mitigation, by which I mean any attempt to lower the concentration of greenhouse gases in the atmosphere, whether by reducing emissions, sequestering CO2, or reforestation, to name only a few possibilities.
[Slide #36: Moving Beyond Current Energy Systems]
(Use "back" to return to the text.)
Moving beyond our current energy systems is surely key to meeting this challenge. To a large extent, 21st-century civilization is still running on 19th-century energy technologies--most notably, combustion of fossil fuels.
And humanity is still practicing a social paradigm unchanged in 100,000 years, that of burying or burning its copious wastes. Only a very small percentage of products are made on the premise that it is better to prevent waste than to clean it up once it is formed—a basic principle of "Green Chemistry" The production of a typical 2 gram computer chip requires about 1.7 kilograms of chemical inputs.5
[Slide #37: Energy Research]
(Use "back" to return to the text.)
In every area of energy development, production, storage, transportation and use, there remain fundamental challenges in basic science and engineering to resolve before we can foresee a future based on sustainable energy. Advancing the knowledge base in all these areas would expand the options for every nation to respond to climate change.
At NSF, our energy research projects branch in many directions--from the examination of chemical catalysts and molecular transformation to innovative studies of wave energy.
And they address fundamental investigations of electron transport, and studies of surface chemistry that could help us along the path to efficient solar energy production.
[Slide #38: New Nano Materials for Hydrogen and CO2 Capture]
(Use "back" to return to the text.)
A broad portfolio of investments seems a good strategy when there is no clear leader in the field. Even investments in fundamental nanoscale science and engineering can bear fruit. For example, NSF-supported investigators at UCLA6 have shown that a class of new materials called metal-organic-frameworks--or MOFs for short--can store large amounts of carbon dioxide under ambient conditions.
This development has the potential to improve the efficiency and lower the costs of carbon capture and storage systems. MOFs also hold promise for hydrogen storage, a critical roadblock to the practical use of hydrogen as a fuel source.
It would be hard to exaggerate the challenges of energy R&D or the need for speedy progress. The magnitude of these challenges was brought home by a recent commentary in Nature magazine.7
According to calculations by the Nature authors, the IPCC assumes that 57 to 96 percent of the total carbon removed from the energy supply will occur through routine technological progress. These assumptions are built into the set of scenarios associated with stabilizing carbon dioxide in the atmosphere. The goalpost is even farther down the field than we thought.
Energy is by no means the only important focus for research and technology development. Innovative technologies could help with coastal erosion, diminishing water supplies, infrastructure and much more.
[Slide #39: Water: A Scarce Resource]
(Use "back" to return to the text.)
These complex issues are interconnected. Consider the links between energy and water, for example. In the U.S., electricity production and agriculture each account for roughly 40 percent of freshwater withdrawals.
[Slide #40: Water Consumption and Energy]
(Use "back" to return to the text.)
The amount of water required to produce energy varies greatly for different systems. As fresh water supplies become scarcer, this will be an increasingly important factor in choosing energy technologies.
Simply stated, without water, there is no life. UNESCO8 reports that by 2025, more than half the nations in the world will face freshwater stress or shortages. By 2050, as much as 75 percent of the world's population could face freshwater scarcity.9
[Slide #41: Water on Planet Earth]
(Use "back" to return to the text.)
Although the salty oceans cover nearly 70 percent of the planet, less than 3 percent of the planet’s total water is fresh. About 30 percent is found in ground water. Rivers, lakes, and clouds carry less than 1 percent. Nearly 70 percent of fresh water resides in ice caps, glaciers, and permanent snow.
[Slide #42: Himalayan Glaciers]
(Use "back" to return to the text.)
Earlier I pointed out the important link between the vast stores of freshwater in the Polar Regions and sea level rise. Scientists have concluded that the rising global temperature is a major contributor to the melting of Himalayan and other tropical glaciers. Mountain snowpack is a large source of water in many regions including India and the western United States. Ice loss from glaciers reached record levels in 2006, and many mountain glaciers could disappear completely within decades.
Investigators recently discovered that pollution-filled "brown clouds" over south Asia enhance solar heating of the lower atmosphere by about 50 percent. The combined heating effect of greenhouse gases and brown clouds, which contain soot, trace metals and other particles from urban, industrial and agricultural sources, is enough to account for the retreat of Himalayan glaciers in the past half century.
The glaciers supply water to major Asian rivers, including the Yangtze, Ganges and Indus. These rivers are the chief water supply for billions of people in China, India and other south Asian countries.
[Slide #43: International Collaboration]
(Use "back" to return to the text.)
The intellectual creativity and capital needed to address the complexities of climate change science, and the engineering challenges of both mitigation and adaptation to climate change, can only come from a concerted international effort.
The research I have mentioned today is funded by NSF. Many of these projects are also supported by other U.S. agencies. As important, many are collaborative efforts involving many international partners, Japan among them. No nation can hope to solve this problem alone.
NSF has long provided support for the climate efforts of the National Center for Atmospheric Research and other scientists working on climate issues. We are proud that these scientists shared in the recent Nobel Prize awarded to the IPCC team. I'm certain that you are equally proud of the many Japanese scientists who participated.
Now we must ponder how to translate this new knowledge into effective policy. It's a challenge to understand--in the words of one technology scholar10 "how intelligent social decisions can be made in the face of great complexity, high uncertainty, and rampant disagreement."
Nevertheless, these are precisely the decisions we must make in the case of climate change. Science and technology can help us make smarter choices by serving up the broadest possible set of options, informed by a robust science and technology base. Our commitment to international collaboration will determine how effective we are in realizing this great potential.
[Slide #44: Earth (with Moon)]
(Use "back" to return to the text.)
"More research" is no substitute for responsive and responsible climate change policies. But remaining uncertainties must be addressed if we are to have any hope of predicting and responding appropriately to climate change. Adequate forecasting, where possible, is central to short- and long-range mitigation and adaptation strategies that are flexible and effective.
The payoff will be greater progress in solving the climate dilemma that confronts societies around the globe.
I offer to you, my friends and colleagues who share these common concerns, a commitment to communicate, cooperate, and collaborate to create a better future.
NOTES

1. David Talbot, "Seeing Greenland," MIT Technology Review, March/April 2007, p. 24 (Return to speech)
2. This area is home to the Pine Island Glacier, one of the most dynamic and fast-moving Antarctic glaciers, which sends more than 69 cubic kilometers of ice per year into the Amundsen Sea. (Return to speech)
3. Crutzen, Paul, "Geology of Mankind," Nature 415, January 3, 2002, p. 23. (Return to speech)
4. Report of the World Commission on Environment and Development: Our Common Future, 1987. (Return to speech)
5. Environ. Sci. Tech. 2002, 36, 5504. (Return to speech)
6. Research conducted by Omar Yaghi, UCLA, supported by NSF and DOE. (Return to speech)
7. "Dangerous Assumption," Nature 452, April 2, 2008, pp. 531-532. The Nature commentary is co-authored by scientists Roger Pielke, Jr., of CU-Boulder, Tom Wigley of NCAR and economist Christopher Green of McGill University. (Return to speech)
8. http://www.unesco.org/water/wwap/wwdr/ (Return to speech)
9. "The Energy Challenge," Mike Hightower and Suzanne A. Pierce, Nature, Vol. 452, March 20, 2008, pp. 285-286. (Return to speech)
10. "Information Technology, Muddling Through, and the Potential Intelligence of Democracy," E. J. Woodhouse, Rensselaer Polytechnic Institute. Last accessed April 2, 2009: http://www.rpi.edu/~woodhe/docs/midwest.htm (Return to speech)
|