Neural Crest Cells: The First Mystery of Craniofacial Development
In 1868, the Swiss embryologist Wilhelm His spotted a thin band of previously undetected cells bunched between fetal ectoderm and the inchoate neural tube of a developing chick. Dr. His called his find the Zwischenstrang, or “the intermediate cord.” By the end of the century, the German word Zwischenstrang had been scrapped for the more descriptive English term “neural crest cells,” denoting the geographic crest of the neural tube as their site of origin. The cells also had become a topic of controversy. Reports had begun to trickle into the scientific literature that neural crest cells in some fish gave rise to neurons and nerve fibers of the cranium, while those in certain salamanders were proposed to produce cartilage of the head and dentin forming cells of the teeth. Many biologists claimed this was preposterous.
“One hundred years ago, claiming that an ectodermal derivative such as the neural crest was in any way involved with the formation of skeletal structures was the embryological and evolutionary equivalent of nailing an additional thesis to the cathedral door....”
“One hundred years ago, claiming that an ectodermal derivative such as the neural crest was in any way involved with the formation of skeletal structures was the embryological and evolutionary equivalent of nailing an additional thesis to the cathedral door,” wrote Langille and Hall in the early 1990s, referring to Martin Luther’s famous Protestant rebellion. “That skeletal structures were mesodermal in origin was dogma, known and accepted by all; an ectodermal origin was heresy.”
Today, the controversy has ebbed. Scientists have solidly established that these short-lived precursor cells come in four distinct types, all of which are programmed to migrate throughout the body and seed new tissue. Among them are the cranial neural crest cells that, as mentioned above, help to generate most of the distinctive skeletal structures of the head and face. Although the mystery of neural crest cells historically has attracted anatomists and evolutionary biologists, the last few decades have brought more molecular and cell biologists to the field. The prospect of increased collaboration among the scientific disciplines, coupled with the rapid progress in research technology, promises to herald a new era of discovery in craniofacial development.
To take a closer look at neural crest cells, craniofacial development, and the research job ahead, two NIDCR grantees offer their thoughts. We start with Dr. Marianne Bronner-Fraser, a biologist at the California Institute of Technology in Pasadena, and Dr. Paul Trainor, a scientist at the Stowers Institute for Medical Research in Kansas City, Missouri.
Marianne Bronner-Fraser
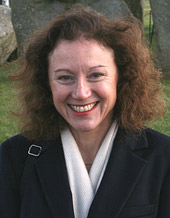
Dr. Marianne Bronner-Fraser
California Institute of Technology
Pasadena, California
In studying craniofacial development, why go all the way back to neural crest cells?
In other words, why study the Book of Genesis? Why not just cut to the Book of Revelations and fully formed human tissues?
Think of it like this. You wouldn’t understand the meaning of Tolstoy’s War and Peace, by flipping to the last few chapters. The same is true here. You’ve got to start as close to the beginning as possible to follow the biological narrative and, hopefully, tease out its underlying molecular logic and morphological patterns.
What are some of the early features of the narrative?
Well, the initial generation of neural crest cells. It’s really quite fascinating. The early embryo forms as three distinct layers of tissue – the exterior ectoderm, the middle mesoderm, and the internal endoderm. By day 19, the interaction of ectoderm and mesoderm produces the neural plate, a precursor of the central nervous system.
And neural crest cells form along the neural plate?
Exactly. The interaction between ectoderm and mesoderm is a classic mode of embryonic tissue formation. That’s why the lessons learned here will have relevance to understanding tissue formation elsewhere in the body. That’s also why it’s essential to define the molecular machinery within the neural crest cells that prompt them to migrate. In other words, which molecular gears and sprockets turn on and off to enable neural crest cells to transition from ectoderm to mesoderm? How does this transition enable them to loosen from the neural tube and migrate, for example, to the heart or the cranium? And, of course, in the context of cranial neural crest cells, it’s essential to understand how these cells at first produce what appears to be the same generic, undifferentiated facial primordial in vertebrate species. And yet, neural crest and the surrounding ectodermal cells generate these dynamic development programs that produce vertebrate structures as distinct as the beak of a toucan, the tusk of a boar, or the venom-producing salivary gland of a rattle snake.
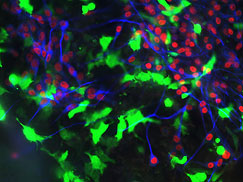
Neural crest cells with placode cells expressing neuronal markers.
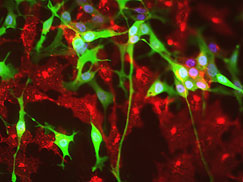
Placode neurons with neural crest cells.
Your group is looking comparatively up and down the evolutionary ladder not only at vertebrates but invertebrates, or more primitive creatures that lack a spinal column and thus a head. Why?
That’s where many of the answers to vertebrate evolution will be found. People tend to be so human centric and, because of that, vertebrate centric. But if you can piece together and understand some of the evolutionary changes that occurred through the millennia from invertebrate to vertebrate, they can be extremely informative in telling us how something as complex as a human head is assembled. In short, we need to listen to the biology, not impose our own mechanistic thoughts and metaphors upon it.
And by listening to the biology, it also will help to explain where things go wrong to cause a malformation, say a cleft lip?
Sure. I think a lot of the answers to birth defects will lie in the early stages of craniofacial development. One of the things that I’m very interested in is the gene regulatory networks in neural crest cells that help to initiate this self-assembly machine that forms a head. You start development in all species with a single-cell fertilized egg. Without giving any obvious hints, that lone cell gives rise to structures and faces as diverse as those of a human being, a finch, and a giraffe.
And yet, as complex as craniofacial development is, it usually is completed without a hitch.
That’s right, craniofacial development goes right in the vast majority of cases. What’s interesting is the same genes that are used early in development also are used later in the process and at multiple times. It seems to be a re-iterative process during which you have important genes that are first used to specify a cell type and then later that same gene might be used to tell it to differentiate into a tooth, bone, or jaw cell. It’s fascinating to realize that the toolkit is not as vast as we once thought it must be. It’s the way that toolkit is deployed that proves to be especially important.
Has the genetic activity of neural crest cells during the developmental process been catalogued?
We’re getting there. With the full genetic complement, or genomes, of various species now determined, the rate of identifying genes involved in making a head or a heart has increased exponentially. It’s left us wading through a huge amount of data. That’s exciting in that so many more pieces to the puzzle are spread out on the table. The problem is this heavy volume makes assembling the puzzle more complex. If I’m dealing with 500 or 1,000 genes to figure out how they work together to create a cell type or render a neural crest cell migratory, I might be really puzzled. If I have a colleague who is looking at it from an opposite approach but happens to identify a subset of those genes, we can take those 1,000 candidate genes and cut them to 10 and begin thinking about communication nodes and signaling networks. What I’m hoping is that by putting these groups together, we can narrow down the key players more quickly. There’s going to be common themes running through this. Unless you do something comparatively, you can’t see those threads.
And these multidisciplinary groups have been formed and continue to be?
That’s right. For example, the NIDCR soon will launch its FaceBase Project. It will bring together scientists of various research backgrounds and establish collaborative consortia that focus on specific sequences of craniofacial development. That will be very constructive. Our best science is ahead of us, and, as more of the metaphorical pieces to the neural-crest puzzle are discovered, I think fitting them into a coherent biological picture of craniofacial development will have profound implications for human health and disease.
Paul Trainor

Dr. Paul Trainor
Stowers Institute for Medical Research
Kansas City, Missouri
Has the push for multidisciplinary research been a positive change in the field?
Oh definitely. I would say that combining disciplines has helped in the last few years to increase the scale and pace of the research in general. You can see that with the NIH Roadmap Initiative. The Roadmap, because of its larger scope, interdisciplinary emphasis, and often the uniqueness of its projects, can open up very rapidly numerous unexpected avenues of study and thus a number of spin off projects. In neural crest research, we’re already benefiting from this progress.
How so?
People no longer look at neural crest cells and facial development from a single-gene perspective. That had long been the norm, largely because of technological limitations. People now have a better set of investigative tools at their disposal and are broadly defining the different gene regulatory changes in neural crest cells. They also are taking the next biological step and defining the protein signaling networks that must be activated for initial crest cell formation, and those that subsequently trigger their three-dimensional patterning. That will also impact our understanding of neural crest cell evolution.
But neural crest cells are only a part of the developmental story. There are also the pharyngeal arches that they colonize and which serve as an inductive template – the facial primordial – for craniofacial development.
That’s right, it’s a two-way street. The neural crest cells certainly don’t exist in a vacuum. Historically, we used to think that neural crest cells did a lot basically by themselves. I’d say that if one thing has changed dramatically in the field over the last five to 10 years, it’s been this recognition that, yes, neural crest cells have species specific programming information. But a lot of what they do is dependent on which tissues they contact during their migration and which signals are received when they reach their final resting place.
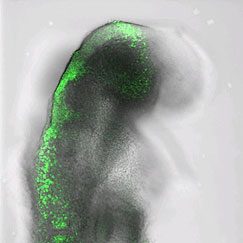
A view of the neural plate and migrating cranial crest cells.
What I’m wondering, though, does the facial primordial serve as the rough blueprint of a vertebrate head?
That may well be. If you look at the different vertebrate species, there are tremendous similarities in the initial formation and migration of neural crest cells into the pharyngeal arches, also called the branchial arches in fish. There’s no doubt about that. But if you fast forward the developmental process and look at the diversity of facial structures that arise across the vertebrate spectrum, they are dramatically different from the trunk of an elephant to the tusks of a boar. So what is highly conserved in nature is this early basic blueprint of facial development, and then the diversification themes that take place beyond that time point.
When you say “diversification themes,” that’s where neural crest cells play such a major role?
Correct. Neural crest cells are endowed with an innate plasticity, or an ability to make developmental modifications. That means the genetic program wired into the neural crest cells don’t need to be modified at the neural tube, that is, the very front end of their life cycle. The modifications can occur throughout the developmental process. By that I mean, cranial neural crest cells can be influenced by molecular factors during their migration to the pharyngeal arches. Or, they might arrive there at a new position, where they have multiple tissue interactions that modify their location, orientation, and ultimately their fates.
And it’s this plasticity that leads to diversity?
That’s right. The plasticity allows such tremendous variation. If everything was rigidly preprogrammed when neural crest cells form in the neural tube, you certainly wouldn’t have the same flexibility. Vertebrate faces might look relatively the same from one species to the next.
When can neural crest cells first be detected during embryonic development?
In the mouse and chick, we’ve gone all the way back to the induction process, which is the earliest relevant time point [embryonic Day 8 in the mouse, 1.5 in the chick]. If we went back any further, we’d see things that might influence neural crest formation secondarily, such as the development of the neural plate. But they may not be particularly relevant to the actual induction process.
So, in these species, you can track neural crest cells from just about point A to Z of the developmental process?
Yes, exactly. What we can’t do is tackle that in humans for technical and ethical reasons. What we do know, if you think about the different phases of neural crest development – whether it be the formation, migration, differentiation phase – if an anomaly arises in any one of those phases, you can end up with a very different craniofacial malformation.
For example?
Well, there a number of recognized neural crest-derived malformation syndromes. I’m talking about potentially devastating conditions such as Treacher-Collins Syndrome or DiGeorge Syndrome. What’s clear is these often severe syndromes arise within the first eight weeks of pregnancy. At the moment, there is no way that we can detect or visualize them in people during these early, in utero stages based on morphology alone. I think an exceptionally skilled sonographer, even if he or she was specially trained to detect craniofacial anomalies, wouldn’t necessarily be able to do it with 100 percent accuracy, even at 22 to 25 weeks of pregnancy.
I should note that even if one could recognize a potential problem, many craniofacial syndromes are quite similar and overlap in their phenotypes, or visible manifestations. They also vary in severity from child to child. So, the precise identification of a specific condition still requires genetic confirmation. It can’t be done visually.
What needs to change to turn back the detection clock?
Well, it’s incredibly complex. I guess one is the development of improved technologies to visualize the very early embryo. But that’s only part of it. I think it is very difficult at that early stage to say whether something looked unusual and needed to be monitored and then to try to figure out what it was that was abnormal. It’s not always so clear.
How will clarity be reached?
I would say the key is building on the recent progress in biology. If we have good mouse models of individual diseases, we can take all of the genetic network information for neural crest patterning, migration, and differentiation and pinpoint the origin of the problem. If it turns out to be something as simple as a wave of cell death – the neural crest cells are being killed off – then the simplistic idea is to find something that will keep them alive, in the same way that some people have found that folic acid somehow confers better viability to neuroepithelial cells and can reduce the incidence of neural tube defects. That’s the approach that we’re taking. We start with a specific syndrome, try to identify its cause, test whether the mechanism may be common to similar syndromes, and see if we can find a way either to chemically or genetically rescue the problem.
But, as you said, nobody will get anywhere without doing the biology?
That’s right. Everyday presents a new challenge, but we enjoy our work and its potential to benefit substantial numbers of people worldwide.
Next: Tooth Development