About LANL
About Our Capabilities, Facilities, and Staff
"Los Alamos National Laboratory plays an indispensable role in building America as a science and technology powerhouse, and our staff are an incredible resource to the nation and the world." Michael Anastasio, Dir.
Solving Complex R&D Problems with Special Blend of Staff, Capabilities and Facilities
Now in its seventh decade, LANL remains among a very few laboratories that can bring great breadth of fundamental and discovery science, technology, and engineering rapidly together to create tangible solutions for national security needs.
Our staff, working with partners throughout science and industry, must be able to deliver today's solutions while maintaining the depth of capabilities to deliver the next generation of discoveries.
Los Alamos has demonstrated a cycle of innovation where we have developed world-leading capabilities and facilities in response to urgent, unique missions. We also spin out new discoveries that lead to emerging missions.
Being able to integrate and apply our capabilities rapidly to new challenges will be a key advantage in an increasingly competitive landscape.
Our Science, Technology and Engineering Priorities
Science that Matters
- Information science and technology enabling integrative and predictive science
- Experimental science focused on materials for the future
- Fundamental forensic science for nuclear, biological, and chemical threats
How We Work
- Collaborate, partner and team to make decisive contributions to our sponsors
- Outstanding operational excellence for safety, security, and efficient pursuit of ST&E for our missions
Transform Our Scientific Campus
- Campus for 2020 (consistent with complex transformation)
- Modern science facilities: LANSCE refurbishment, CMR replacement, Science Complex
- Signature facilities for experimental science (MaRIE) and computational science (Roadrunner)
More About This Science
Promising New Characterization of Super-cooled Materials
Scaling the Kondo Lattice
Provides insight to superconductivity and power generation

Quick read
Tremendous improvements in electricity transmission and storage are on the horizon, thanks to studies of the Kondo lattice, a configuration of electrons that appears in certain super-cooled materials.
Scientists from Los Alamos National Laboratory have proposed a new characterization for the bizarre behavior of certain super-cooled materials that could dramatically change power generation and storage.
The researchers' work was published in the renowned journal Nature in July.
Superconductivity, in which electrons flow through a system without resistance, holds great promise if it can be accomplished at high temperatures. It could mean tremendous energy efficiency in such applications as the transmission of electricity and electric motors for mass-transit trains. Superconducting magnets are currently used in Magnetic Resonance Imaging (MRI) machines in hospitals, but for many applications, superconductivity is too expensive to be practical. For the phenomenon to occur, the material must be cooled to several hundred degrees below zero, Celsius, often by means of expensive chemicals, such as liquid nitrogen and helium.
The mysterious behavior of electrons in what's known as the Kondo lattice, a material with a trellis-like network of localized electron spins embedded in a sea of mobile electrons, has perplexed physicists for years. In these compounds, localized electrons and mobile electrons behave independently near room temperatures but change their character dramatically at very low specific temperatures as a result of the collective entanglement of the localized spins with the mobile electrons at the subatomic level.
At this low temperature, a new state of matter emerges in which the mobile electrons gain weight as the local electron spins lose their magnetism. One signal of the onset of this new "heavy-electron" state (known as a Kondo liquid) is a specific change to the electrical resistance of these materials.
In ordinary metals, electrical resistance decreases as compounds get colder, but for these materials, resistance first increases as a result of the scattering of the conduction electrons against the localized electrons; then, as these electrons' interactions lose their strength, the resistivity starts to decrease.
"The previous understanding was to see this behavior as a lattice extension of what happens when an impurity is present in a compound," says lead author Yi-Feng Yang, a postdoctoral scholar at Los Alamos and UC Davis. "Our paper shows that's not the case."
The Kondo-lattice temperature, the unique critical temperature below which the electrons in the Kondo lattice begin to develop their quantum-entangled state, is shown to be quite distinct from, and much larger than, the characteristic temperature at which a single, localized-impurity electron spin becomes entangled with a mobile electron sea. The authors show, however, that an unexpectedly simple relationship exists between these two characteristic temperatures: Both temperatures depend on a single variable that measures the strength of the interactions between local spins and mobile electrons.
"This body of work really takes the study of heavy electrons from stamp collecting into a science because now you have a unified framework for looking at all these materials," says UC Davis physicist and coauthor David Pines.
Instead of focusing on each individual electron-electron interaction, as a collector might haphazardly pick up stamps from various countries around the world, scientists can now quantify the underlying reasons for this mystifying behavior in existing heavy-electron materials and predict the behavior of newly discovered members of this family.
Since the 1970s scientists have relied on the Doniach diagram, which attempts to explain this complex behavior on an either-or basis: either local spin behavior dominates, leading to antiferromagnetism, in which the compound loses all net magnetism, or mobile electron behavior dominates, with the possibility that the material becomes superconducting. The new approach suggests this competition occurs between two quantum-ordered states of the heavy, but mobile, Kondo liquid.
"In the same sense that Doniach's ideas have been influential for the past 30 years, it's possible that this [paper] could influence our understanding of these materials for the coming 30 years," said Joe Thompson, a Los Alamos physicist and coauthor.
As deeper knowledge of the mechanisms emerges, it may be possible to drive the temperature even higher, perhaps to room temperature, the "Holy Grail" of superconducting temperatures because it would not require refrigeration. Scientists could even begin creating new forms of matter.
Los Alamos researchers collaborated on this project with the University of California, Irvine, and the University of California, Davis. Also coauthors on the paper were Han-Oh Lee of Los Alamos National Laboratory and Zachary Fisk of the University of California, Irvine.
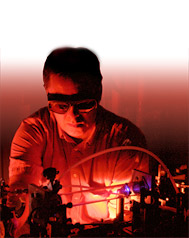
Table-top lasers compete with large accelerators
George Rodriguez adjusts argon gas pressure inside two-color plasma ionization gas cells
Table-top ultrafast laser based plasma terahertz sources are beginning to rival pulse energies previously obtainable only at large accelerator-based facilities.
Currents, the Laboratory's monthly employee magazine, highlighting people in the workplace.