PODCASTS ABOUT
PODCASTS ABOUT
RSS
Research in NOAA
Interview with Harold Brooks
November 8, 2007
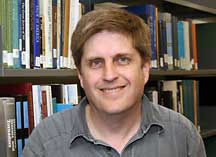
BARRY REICHENBAUGH: This is Barry Reichenbaugh. I'm with the NOAA
Research Communications Office here in Norman, Oklahoma. And I'm with
Harold Brooks. Harold, could you give us your title?
HAROLD BROOKS: Sure. I'm Harold Brooks. I'm a research
meteorologist with NOAA's National Severe Storms Laboratory in Norman,
Oklahoma.
BARRY REICHENBAUGH: Harold, your area of expertise is
tornadoes. Can you go into a little bit about when and where tornadoes
occur?
HAROLD BROOKS: Tornadoes have been observed in every
state of the United States and in all the continents of the world except
for Antarctica. And they have occurred any time throughout the year
in the U.S. Now, most tornadoes occur in the late afternoon, in the
springtime, and primarily in the region between the Rocky Mountains and
the Appalachian Mountains.
BARRY REICHENBAUGH: What are the risks in different parts
of the country?
HAROLD BROOKS: If we try to think about why tornadoes
occur, we need to step back and think about, Well, what are the ingredients
that we need to have in the atmosphere to make a tornado? And to start
with that, we actually think about what kind of ingredients we need for
a thunderstorm, because tornadoes come from thunderstorms.
If we think about the ingredients we need for a thunderstorm,
we need basically three things. We want to have warm, moist air at the
low levels near the ground. We want to have relatively cool, dry air
aloft, and then some mechanism to lift that warm, moist air up. And
as that moist air goes up, it cools off less rapidly than the air around
it, then it actually becomes warmer than the air, less dense, and so
it rises just like a hot air balloon.
So we need those three things to have a thunderstorm at
all. And to get the kind of storm that will make a tornado, we need
to have the winds increase in strength from the ground as you go up into
the atmosphere and typically change direction. Usually out of the south
at low levels and out of the west aloft.
And so when we think about how things work in the United
States, it's pretty easy to see why the central part of the country gets
more tornadoes than any other place. If we have air at low levels coming
out of the south, that air is coming from off the Gulf of Mexico, bringing
in lots of the warm, moist air. If we have air aloft coming from out
of the west, it's coming from over the Rocky Mountains. And the best
way you can get dry air aloft in the atmosphere is to lift it up over
a wide, high range of mountains. And the Rocky Mountains are the perfect
kind of place to do that.
And so when we bring the ingredients together of the warm,
moist air at low levels and the dry air aloft, in the middle part of
the U.S. we typically have the air out of the south at low levels, out
of the west at high levels, and that brings everything together to make
the potential for tornadic thunderstorms.
And, in fact, the central part of the United States is
probably the perfect laboratory on earth to produce those kinds of conditions
more often than any other place. If we look at the other places that
get tornadoes frequently, there's at least one of the ingredients that
it's really hard to bring together. And so it just happens a lot more
often in the central part of the U.S.
Now in the wintertime, the atmosphere, in effect, has
to work a little bit harder. It has to bring the ingredients together
with larger-scale conditions happening, and that tends to happen more
often in the southeastern part of the United States -- say, Alabama,
Georgia, Florida -- in wintertime. The southeastern part of the United
States has a problem with tornadoes in a different way than the central
part of the U.S. To get the ingredients together, the atmosphere has
to, in effect, work a lot harder. It has to bring large-scale conditions
together to bring the ingredients into the same place at one time.
As a result the seasonal cycle, where we have the very
strong peak in the springtime and maybe in the early summer in the central
part of the U.S., we don't see that in the southeast. The seasonal cycle
is a lot less distinct, and it actually has a lot of tornadoes happening
in what we call the "cool season," sort of November to March.
And they also tend to get more tornadoes occurring after
dark in the southeastern part of the United States, because the atmosphere
doesn't really care about the diurnal cycle in that part of the country
as much as it does in the central part of the country. And so while
in the central part of the U.S., our threat tends to be late spring and
typically late afternoon, early evening, in the southeastern part of
the U.S., there's not so much of a distinct timing mechanism with the
threat.
BARRY REICHENBAUGH: Okay. You've talked some about the
regions that tend to get tornadoes. Can you talk a little bit about
Tornado Alley and why we use that term?
HAROLD BROOKS: Okay. Well, Tornado Alley is one of those
terms that if you ask 20 people to study the subject, you'll probably
get 20 different definitions.
We tend to think of it as perhaps including the region
that gets the most tornadoes, but also perhaps we get them kind of regularly.Â
You can, kind of, count on the fact that every year is going to be a
pretty good year. If we just think about the part of the country that
gets the most tornadoes, we'd probably draw something that looks kind
of like an L or a C shape maybe. That starts with the bottom left part
of the letter around Dallas, Texas, over to Midland, Texas, and then
maybe up through the High Plains, up into Nebraska, maybe South Dakota.
The bottom part of the L would extend perhaps into Mississippi
or Alabama, and the top part goes over towards Ohio. Now, if we want
to include information about the fact that tornadoes happen almost every
year and regularly, then we probably lose the eastern part of that extension,
and maybe we only go as far east as Tulsa or the Oklahoma/Arkansas border,
where we get a lot of tornadoes in that region that goes from Midland
to Dallas and north for several hundred miles.
We get a lot of tornadoes in there, and we also have them
almost every year. Most places will have a tornado come pretty close
to them.
BARRY REICHENBAUGH: Let's get a little bit into how we
communicate the risk, and maybe if you could talk a little bit about
this Enhanced Fujita Scale.
HAROLD BROOKS: Good. Well, one of the real challenges
we have in studying tornadoes is that we'd like to be able to describe
how strong a tornado is. We know that not all tornadoes are the same,
but that we don't really get wind measurements very often. It's a very
different problem than you have when you look at hurricanes. We fly
aircraft into the hurricanes. The hurricanes are over a fairly large
area, and so there's a lot of surface stations that collect data.
The chances of a tornado hitting an anemometer are really
small, and while we have some mobile radars that can go out and collect
information inside the tornado, we still don't see very many tornadoes
that way. Maybe 20 or 30 a year out of the 1,200 that happen every year
in the United States, so we don't have a very big sample.
So in order to estimate how strong the winds are in a
tornado, we have to look at what damage the tornado causes. And to do
that there have been some scales that have been set up to try to look
at that, that basically start by saying, Well, how much damage did the
tornado itself do?
And the oldest and longest serving of those was developed
by Ted Fujita at the University of Chicago in the early 1970s. And for
years the National Weather Service rated all tornadoes on the Fujita
Scale, which was based upon what kind of damage would be caused to a
well-built frame home. Well, that causes some problems, because if you're
an engineer, you look at the house and you go, Well, that house, maybe,
wasn't really well built; or, This other house was really well built.Â
And so maybe the differences we see in the winds are because, actually,
the houses were built differently. If the house wasn't attached to the
ground very well, then it might fall apart easily.
So in 2007 the National Weather Service, after a several-year
project to try to improve the Fujita Scale, brought out what was called
the Enhanced Fujita Scale, which instead of basing the damage off of
just one kind of thing, the well-built frame home, now it uses a large
number of damage indicators and a large number of degrees of damage.Â
Was the house barely hit or was it completely destroyed? Were the shingles
taken off? Was it a gasoline station awning that was removed?
And so now damage estimators can go out and have a much
broader range of techniques to try to apply to it. Now, it still needs,
probably, some improvement, because it was not an engineering rated study;
in other words, it wasn't the kind of thing that engineers sat down and
wrote equations out and said, Here's the amount of force it takes to
blow this object down. And so we still have some issues about exactly
how to do it. And so hopefully it'll be improved as it goes along.Â
But it does allow estimators a much broader range of information to use
when they try to estimate the wind speeds.
BARRY REICHENBAUGH: Let's touch on some general information
about some tornadoes, what we know and what we don't know.
HAROLD BROOKS: Sure. Okay. Well, the biggest thing
we know about tornadoes is the kinds of storms that'll make the strongest
tornadoes. We call them supercell thunderstorms. And in fact in a supercell,
the updraft of the storm, the part of the air that's going up, is in
fact rotating throughout the entire depth of the storm, from just above
the ground maybe up to 50,000 feet above the ground.
And that's one of the things that makes them an attractive
target for radars, is because with Doppler radar, we can see the motions
inside that storm. So they've been studied for a long time. And although
we don't have precise numbers, it certainly looks like that almost all
of the strong tornadoes, the ones that will actually cause damage to
a house, come out of supercell thunderstorms. And so they become a particularly
important kind of a problem.
And we understand the large-scale conditions that lead
to the development of those kinds of storms. Something we don't understand
quite so well is what makes a supercell produce a tornado, versus a supercell
that doesn't make a tornado. And we have a pretty good idea of the kinds
of conditions that lead to that. We need to look at things that are
happening very near the ground, both in the environment of the storm
and within the storm itself. And that makes them harder to study, because
now instead of being able to look over this large depth of the storm
and a large depth of the atmosphere, we have to have really precise kinds
of measurements down there in the very lowest part of the atmosphere.
The second thing we don't really know is exactly what
happens in the last few minutes before the storm makes a tornado. The
time between when some things start to look like they're potentially
tornadic until the storm actually makes a tornado can vary from a few
minutes up to half an hour or more within a storm. And so that's a real
problematic aspect.
We also don't know why tornadoes die. In many ways it's
easier to understand how a tornado starts than how it actually ends.Â
And it would be a big help for warning forecasters if we actually start
to predict, Well, this tornado's about to end. Then you wouldn't have
to put a warning out for, say, the next county, because you knew that
the tornado wasn't going to be happening.
And I think the final thing that we don't really understand
is why tornadoes are as intense as they are. It's very difficult to
know, watching a storm, Will this storm make a very strong tornado or
will it make a weak tornado? And in fact even knowing what other tornadoes
a storm has produced, if it's producing more than one, you don't always
know how strong that next tornado is going to be. And if we could actually
learn that process that determines the intensity of the tornado, then
we might be able to tailor the warning statements to be able to say,
Well, this storm is particularly dangerous. And you really, really need
to be doing very special things to protect yourself. Or, This is going
to be a relatively weak tornado, and if you're inside a well-built home,
the wind's going to blow pretty hard and you might get a window broken,
but there's not a real big danger to your life.
BARRY REICHENBAUGH: Sounds like there's still room for
some more research.
HAROLD BROOKS: Oh, there's still lots of room for research.Â
We don't theoretically understand everything that goes on in the tornado
particularly well, and we know that we have to do a lot better job with
the observational things. I think the biggest thing that we've learned
in the last 15 years is that the kinds of questions we ask now are much
more detailed than the questions we asked 15 years ago. And if you go
back even further, if you go back, say, 40 years ago, we weren't even
sure what part of the thunderstorm tornadoes came out of. Now we're
asking really narrow questions.
BARRY REICHENBAUGH: Tell me how you got into this field.Â
Where did you get the inspiration?
HAROLD BROOKS: Well, I kind of stumbled into meteorology
in an offhand way. I was always interested in science and math, and
those were always, I thought, the easiest subjects possible through school.Â
And when I was in college I was a Physics and Math major. And as I was
looking around for what I was going to do, I wasn't real thrilled about
what I saw as the job prospects and exciting topics in physics; they
didn't match up with what I thought was the fun part of physics.
And in my junior year in college, my undergraduate department
chair said, ‘You really ought to do something over the summer. This
summer after your junior year, before you go to graduate school, try
something out.’ And he suggested that I go to the Summer Institutes
on planet and climate at the NASA Goddard Institute for Space Studies
in New York. A previous graduate of our college had gone there, had
become a world's expert on black holes, and he said, ‘You ought to go
there. It really helped Don a lot.’
And so I decided, ‘Well, that sounds like a good idea.’Â
And I went to New York and spent the summer there and actually worked
on climate modeling. I put in the boundary conditions for modeling the
last glacial maximum, the last ice age, into a climate model and ran
those. And then Columbia invited me to come back for graduate school,
and so I went back and we had discussed whether I was going to do it
in physics or in geology. And they said the geology department looked
like it was a better fit, and so I actually looked at how volcanic aerosols
are moved in the atmosphere.
And so what happens is if a volcano erupts, what's the
strongest signal it has on cooling the earth if you -- you know, where's
the best place to put it? And after a few years of that I wasn't completely
comfortable with what I was doing right then. I'd grown up in the Midwest
and thunderstorms were always a big part of the summer fun.
And I had the opportunity to come to the University of
Illinois with Bob Wilhelmson, who is probably the leading person in the
world on the numerical modeling of thunderstorms. And that sounded like
a fun thing to do. And Bob and I were probably about as good of a match
of advisor and advisee as you could hope to have. We got along great.Â
Did some work on modeling of why some storms make tornadoes and others
don't make tornadoes.
And then I actually came to Norman simply because of Chuck
Doswell, who was a scientist here at the Severe Storms Lab. At a conference
I had been interacting some with forecasters, and there aren't a whole
lot of researchers that actually like to spend time with forecasters,
because forecasters and researchers think really differently. And Chuck
said, ‘Well, before you go off and get your academic job, why don't you
come to Norman, come to the Lab for a year on a post-doc and come do
some things.’ And the post-doc was a two-year opportunity, and so I
came down for the two years and was fortunate enough to be offered a
federal position when I got done with that, because I decided that interacting
with forecasters and doing those kinds of questions was actually a whole
lot more fun than what I would have in the academic world. And so I've
now been at the Severe Storms Lab for over 15 years as a research meteorologist.Â
I think it's been just a ton of fun.
I talked with friends who seem to have a lot more job
stress than I do. I have to deal with things like a few little bureaucratic/management
things that I have to do that take about 5 percent of my time that aren't
much fun. But the rest of the time I get to work on whatever I want
to and work on things that I think are important and interesting.
BARRY REICHENBAUGH: What do you say to a person who's
considering a career in science?
HAROLD BROOKS: Well, I think one of the things about
science that's a lot of fun is you get to solve problems. You constantly
get to think about things that you want to think about and to try to
find answers for things.
And for any job it's important to actually enjoy what
you do, because otherwise you're pretty miserable. And I think one of
the really fun things about science is the notion of discovery and that
at some point, every scientist gets the excitement of realizing that
they know something that no one else on the planet knows, and then they
get to tell people. And that's just a whole lot of fun.
When you realize something's true that no one's ever known
before, and then you get to stand up and tell people about it or write
about it. And people go, Wow, you learned something important. And
that's just a whole lot of fun. And it happens fairly regularly.