 |
|
Chapter 2
The Fundamentals of Mental Health
and Mental Illness
A vast body of research on mental health and, to an even greater extent, on mental illness constitutes the foundation of this Surgeon General’s report. To understand and better appreciate the content of the chapters that follow, readers outside the mental health field may desire some background information. Thus, this chapter furnishes a
“primer” on topics that the report addresses.
The chapter begins with an overview of research under way today that is focused on the neuroscience of mental health. Modern integrative neuroscience offers a means of linking research on broad
“systems level” aspects of brain function with the remarkably detailed tools and findings of molecular biology. The report begins with a discussion of the brain because it is central to what makes us human and provides an understanding of mental health and mental illness. All of human behavior is mediated by the brain. Consider, for example, a memory that most people have from childhood—that of learning to ride a bicycle with the help of a parent or friend. The fear of falling, the anxiety of lack of control, the reassurances of a loved one, and the final liberating experience of mastery and a newly extended universe create an unforgettable combination. For some, the memories are not good ones: falling and being chased by dogs have left marks of anxiety and fear that may last a lifetime. Science is revealing how the skill learning, emotional overtones, and memories of such experiences are put together physically in the brain. The brain and mind are two sides of the same coin. Mind is not possible without the remarkable physical complexity that is built into the brain, but, in addition, the physical complexity of the brain is useless without the sculpting that environment, experience, and thought itself provides. Thus the brain is now known to be physically shaped by contributions from our genes and our experience, working together. This strengthens the view that mental disorders are both caused and can be treated by biological and experiential processes, working together. This understanding has emerged from the breathtaking progress in modern neuroscience that has begun to integrate knowledge from biological and behavioral sciences.
An overview of mental illness follows the section on modern integrative brain science. The section highlights topics including symptoms, diagnosis, epidemiology (i.e., research having to do with the distribution and determinants of mental disorders in population groups, including various racial and ethnic minority groups), and cost, all of which are discussed in greater and more pointed detail in the chapters that follow. Etiology is the study of the origins and causes of disease, and that section reviews research that is seeking to define, with ever greater precision, the causes of mental disorders. As will be seen, etiology research examines fundamental biological, behavioral, and sociocultural processes, as well as a necessarily broad array of life events. The section on development of temperament reveals how mental health science has attempted over much of the past century to understand how biological, psychological, and sociocultural factors meld in health as well as in illness. The chapter then reviews research approaches to the prevention and treatment of mental disorders and provides an overview of mental health services and their delivery. Final sections cover the growing influence on the mental health field of the need for attention to cultural diversity, the importance of the consumer movement, and new optimism about recovery from mental illness—that is, the possibility of recovering one’s life.
The Neuroscience of Mental Health1
Complexity of the Brain I: Structural
As befits the organ of the mind, the human brain is the most complex structure ever investigated by our science. The brain contains approximately 100 billion nerve cells, or neurons, and many more supporting cells, or
ganglia. In and of themselves, the number of cells in this 3-pound organ reveal little of its complexity. Yet most organs in the body are composed of only a handful of cell types; the brain, in contrast, has literally thousands of different kinds of neurons, each distinct in terms of its chemistry, shape, and connections (Figure 2-1 depicts the structural variety of neurons). To illustrate, one careful, recent investigation of a kind of interneuron that is a small local circuit neuron in the retina, called the amacrine cell, found no less than 23 identifiable types.
But this is only the beginning of the brain’s complexity.
The workings of the brain depend on the ability of nerve cells to communicate with each other. Communication occurs at small, specialized structures called synapses. The synapse typically has two parts. One is a specialized presynaptic structure on a terminal portion of the sending neuron that contains packets of
signaling chemicals, or neurotransmitters. The second is a postsynaptic structure on the dendrites of the receiving neuron that has receptors for the neurotransmitter molecules.
The typical neuron has a cell body, which contains the genetic material, and much of the cell’s energy-producing machinery. Emanating from the cell body are dendrites, branches that are the most important receptive surface of the cell for communication. The dendrites of neurons can assume a great many shapes and sizes, all relevant to the way in which incoming messages are processed. The output of neurons is carried along what is usually a single branch called the axon. It is down this part of the neuron that signals are transmitted out to the next neuron. At its end, the axon may branch into many terminals. (Figure 2-2.)
The usual form of communication involves electrical signals that travel within neurons, giving rise to chemical signals that diffuse, or cross, synapses, which in turn give rise to new electrical signals in the postsynaptic neuron. Each neuron, on average, makes more than 1,000 synaptic connections with other neurons. One type of cell—a Purkinje cell—may make between 100,000 and 200,000 connections with other neurons. In aggregate, there may be between 100 trillion and a quadrillion synapses in the brain. These synapses are far from random. Within each region of the brain, there is an exquisite architecture consisting of layers and other anatomic substructures in which synaptic connections are formed. Ultimately, the pattern of synaptic connections gives rise to what are called circuits in the brain. At the integrative level, large- and small-scale circuits are the substrates of behavior and of mental life. One of the most awe-inspiring mysteries of brain science is how neuronal activity within circuits gives rise to behavior and, even, consciousness.
The complexity of the brain is such that a single neuron may be part of more than one circuit. The organization of circuits in the brain reveals that the brain is a massively parallel, distributed information processor. For example, the circuits involved in vision receive information from the retina. After initial processing, these circuits analyze information into different streams, so that there is one stream of information describing what the visual object is, and another stream is concerned with where the object is in space. The information stream having to do with the identity of the object is actually broken down into several more refined parallel streams. One, for example, analyzes shape while another analyzes color. Ultimately, the visual world is resynthesized with information about the tactile world, and the auditory world, with information from memory, and with emotional coloration. The massively parallel design is a great pattern recognizer and very tolerant of failure in individual elements. This is why a brain of neurons is still a better and longer-lasting information processor than a computer.
The specific connectivity of circuits is, to some degree, stereotyped, or set in expected patterns within the brain, leading to the notion that certain places in the brain are specialized for certain functions (Figure 2-3). Thus, the cerebral cortex, the mantle of neurons with its enormous surface area increased by outpouchings, called gyri, and indentations, called sulci, can be functionally subdivided. The back portion of the cerebral cortex (i.e., the occipital lobe), for example, is involved in the initial stages of visual processing. Just behind the central sulcus is the part of the cerebral cortex involved in the processing of tactile information (i.e., parietal lobe). Just in front of the central sulcus is a part of the cerebral cortex involved in motor behavior (frontal lobe). In the front of the brain is a region called the prefrontal cortex, which is involved with some of the highest integrated functions of the human being, including the ability to plan and to integrate cognitive and emotional streams of information.
Beneath the cortex are enormous numbers of axons sheathed in the insulating substance, myelin. This subcortical “white matter,” so named because of its appearance on freshly cut brain sections, surrounds deep aggregations of neurons, or
“gray matter,” which, like the cortex, appears gray because of the presence of neuronal cell bodies. It is within this gray matter that the brain processes information. The white matter is akin to wiring that conveys information from one region to another. Gray matter regions include the basal ganglia, the part of the brain that is involved in the initiation of motion and thus profoundly affected in Parkinson’s disease, but that is also involved in the integration of motivational states and, thus, a substrate of addictive disorders. Other important gray matter structures in the brain include the amygdala and the hippocampus. The amygdala is involved in the assignment of emotional meaning to events and objects, and it appears to play a special role in aversive, or negative, emotions such as fear. The hippocampus includes, among its many functions, responsibility for initially encoding and consolidating explicit or episodic memories of persons, places, and things.
In summary, the organization of the brain at the cellular level involves many thousands of distinct kinds of neurons. At a higher integrative level, these neurons form circuits for information processing determined by their patterns of synaptic connections. The organization of these parallel distributed circuits results in the specialization of different geographic regions of the brain for different functions. It is important to state at this point, however, that, especially in younger individuals, damage to a particular brain region may yield adaptations that permit circuits spared the damage and, therefore, other regions of the brain, to pick up some of the functions that would otherwise have been lost.
Figure 2-1. Structural variety of neurons
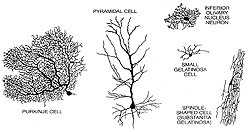
Click to enlarge
Source: Fischbach, 1992, p. 53. (Permission granted: Patricia J. Wynne.)
Figure 2-2. How neurons communicate
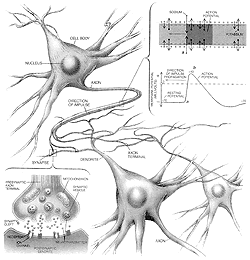
Click to enlarge
Source: Fischbach, 1992, p. 52. (Permission granted: Tomo Narashima.)
Figure 2-3. The brain: Organ of the mind
Source: Fischbach, 1992, p. 51. (Permission granted: Carol Donner.)
Complexity of the Brain II: Neurochemical
Superimposed on this breathtaking structural complexity is the chemical complexity of the brain. As described above, electrical signals within neurons are converted at synapses into chemical signals which then elicit electrical signals on the other side of the synapse. These chemical signals are molecules called neurotransmitters. There are two major kinds of molecules that serve the function of neurotransmitters: small molecules, some quite well known, with names such as dopamine, serotonin, or norepinephrine, and larger molecules, which are essentially protein chains, called peptides. These include the endogenous opiates, Substance P, and corticotropin releasing factor (CRF), among others. All told, there appear to be more than 100 different neurotransmitters in the brain (Table 2-1 contains a selected list).
A neurotransmitter can elicit a biological effect in the postsynaptic neuron by binding to a protein called a neurotransmitter receptor. Its job is to pass the information contained in the neurotransmitter message from the synapse to the inside of the receiving cell. It appears that almost every known neurotransmitter has more than one different kind of receptor that can confer rather different signals on the receiving neuron. Dopamine has 5 known neurotransmitter receptors; serotonin has at least 14.
Table 2-1. Selected neurotransmitters important in psychopharmacology
Excitatory amino acid
Glutamate
Inhibitory amino acids
Gamma aminobutyric acid
Glycine
Monoamines and related neurotransmitters
Norepinephrine
Dopamine
Serotonin
Histamine
Acetylcholine (quarternary amine)
Purine
Adenosine
Neuropeptides
Opioids
Enkephalins
Beta-endorphin
Dynorphin
Tachykinin
Substance P
Hypothalamic-releasing factors
Corticotropin-releasing hormone
|
Although there are many kinds of receptors with many different signaling functions, we can divide most neurotransmitter receptors into two general classes. One class of neurotransmitter receptor is called a ligand-gated channel, where
“ligand” simply means a molecule (i.e., a neurotransmitter) that binds to a receptor. When neurotransmitters interact with this kind of receptor, a pore within the receptor molecule itself is opened and positive or negative charges enter the cell. The entry of positive charge may activate additional ion channels that allow more positive charge to enter. At a certain threshold, this causes a cell to fire an action potential—an electrical event that leads ultimately to the release of neurotransmitter. By definition, therefore, receptors that admit positive charge are excitatory neurotransmitter receptors. The classic excitatory neurotransmitter receptors in the brain utilize the excitatory amino acids glutamate and, to a lesser degree, aspartate as neurotransmitters. Conversely, inhibitory neurotransmitters act by permitting negative charges into the cell, taking the cell farther away from firing. The classic inhibitory neurotransmitters in the brain are the amino acids gamma amino butyric acid, or GABA, and, to a lesser degree, glycine.
Most of the other neurotransmitters in the brain, such as dopamine, serotonin, and norepinephrine, and all of the many neuropeptides constitute the second major class. These are neither precisely excitatory nor inhibitory but rather act to produce complex biochemical changes in the receiving cell. Their receptors do not contain intrinsic ion pores but rather interact with signaling proteins, called“G proteins” found inside the cell membrane. These receptors thus are called G protein-linked receptors. The details are less important than understanding the general scheme. Stimulation of G protein-linked receptors alters the way in which receiving neurons can process subsequent signals from glutamate or GABA. To use a metaphor of a musical instrument, if glutamate, the excitatory neurotransmitter, is puffing wind into a flute or clarinet, it is the modulatory neurotransmitters such as dopamine or serotonin that might be seen as playing the keys and, thus, altering the melody via G protein-linked receptors.
The architecture of these systems drives home this point. The precise brain circuits that carry specific information about the world and that are involved in precise point-to-point communication within the brain use excitatory or inhibitory neurotransmission. Examples of such circuits, which are massively parallel, can be found in the visual and auditory cortex. Overlying this pattern of precise, rapid (timing in the range of milliseconds) neurotransmission are the modulatory systems in the brain that use norepinephrine, serotonin, and dopamine. In each case, the neurotransmitter in question is made by a very small number of nerve cells clustered in a limited number of areas in the brain. Of the hundred billion neurons in the brain, only about 500,000, for example, make dopamine—that is, for every 200,000 cells in the brain, only one makes dopamine. Even fewer make norepinephrine. The cell bodies of the dopamine neurons are clustered in a few brain regions, most importantly, regions deep in the brain, in the midbrain, called the substantia nigra, and the ventral tegmental area. Norepinephrine neurons are made in the nucleus locus coeruleus even farther down in the brain stem in a structure called the pons. Serotonin is made by a somewhat larger number of nuclei but, still, not by many cells. Nuclei called the raphe nuclei spread along the brain stem. While each of these neurotransmitters is made by a small number of neurons with clustered cell bodies, each sends its axons branching throughout the brain, so that in each case a very small number of neurons, which largely appear to fire in unison when excited, influence almost the entire brain. This is not the picture of systems that are communicating precise bits of information about the world but rather are intrinsic modulatory systems that act via other G protein-linked receptors to alter the overall responsiveness of the brain. These neurotransmitters are responsible for brain states such as degree of arousal, ability to pay attention, and for putting emotional color or significance on top of cold cognitive information provided by precise glutaminergic circuits. It is no wonder that these modulatory neurotransmitters and their receptors are critical targets of medications used to treat mental disorders—for example, the antidepressant and antipsychotic drugs—and also are the targets of drugs of abuse.
Complexity of the Brain III: Plasticity
The preceding paragraphs have illustrated the chemical and anatomic structure of the brain and, in so doing, provided some picture of its complexity as well as some picture of its function. The crowning complexity of the brain, however, is that it is not static. The brain is always changing. People learn so much and have so many distinct types of memory: conscious, episodic memory of the sort that is encoded initially in the hippocampus; memory of motor programs or procedures that are encoded in the striatum; emotional memories that can initiate physiologic and behaviorally adaptive repertoires encoded, for example, in the amygdala; and many other kinds. Every time a person learns something new, whether it is conscious or unconscious, that experience alters the structure of the brain. Thus, neurotransmission in itself not only contains current information but alters subsequent neurotransmission if it occurs with the right intensity and the right pattern. Experience that is salient enough to cause memory creates new synaptic connections, prunes away old ones, and strengthens or weakens existing ones. Similarly, experiences as diverse as stress, substance abuse, or disease can kill neurons, and current data suggest that new neurons continue to develop even in adult brains, where they help to incorporate new memories. The end result is that information is now routed over an altered circuit. Many of these changes are long-lived, even permanent. It is in this way that a person can look back 10 or 20 or 50 years and remember family, a home or school room, or friends. The general theme is that to really understand the kind of memory—indeed, any brain function—one must think at least at two levels: one, the level of molecular and cellular alterations that are responsible for remodeling synapses, and, two, the level of information content and behavior which circuits and synapses serve.
To summarize this section, scientists are truly beginning to learn about the structure and function of the brain. Its awe-inspiring complexity is fully consistent with the fact that it supports all behavior and mental life. Implied in the foregoing, is the fact that brains are built not only by genes—and again, it is the lion’s share of the 80,000 or so human genes that are involved in building a structure so complex as the brain. Genes are not by themselves the whole story. Brains are built and changed through life through the interaction of genes with environment, including experience. It is true that a set of genes might create repetitive multiples of one type of unit, yet the brain appears far more complex than that. It stands to reason that if 50,000 or 60,000 genes are involved in building a brain that may have 100 trillion or a quadrillion synapses, additional information is needed, and that information comes from the environment. It is this fundamental realization that is beginning to permit an understanding of how treatment of mental disorders works—whether in the form of a somatic intervention such as a medication, or a psychological
“talk” therapy—by actually changing the brain.
Imaging the Brain
There are many exciting developments in brain science. Of great relevance to the study of mental function and mental illness is the ability to image the activity of the living human brain with technologies developed in recent decades, such as positron emission tomography scanning or functional magnetic resonance imaging. Such approaches can exploit surrogates of neuronal firing such as blood flow and blood oxygenation to provide maps of activity. As science learns more about brain circuitry and learns more from cognitive and affective neuroscience about how to activate and examine the function of particular brain circuits, differences between health and illness in the function of particular circuits certainly will become evident. We will be able to see the action of psychotropic drugs and, perhaps most exciting, we will be able to see the impact of that special kind of learning called psychotherapy, which works after all because it works on the brain.
Different brain chemicals, brain receptors, and brain structures will come up in the discussion of particular illnesses throughout this document. This section is meant to provide a panoramic, not a detailed, introduction and also to provide certain overarching lessons. When something is referred to as biological or brain-based, that is not shorthand for saying it is genetic and, thus, predetermined; similarly, references to “psychological” or even
“social” phenomena do not exclude biological processes. The brain is the great integrator, bringing together genes and environment. The study of the brain requires reducing problems initially to bite-sized bits that will allow investigators to learn something, but ultimately, the agenda of neuroscience is not reductionist; the goal is to understand behavior, not to put blinders on and try to explain it away. As the foregoing discussion illustrates, the brain also is complex. Thus, having a disease that affects one or even many critical circuits does not overthrow, except in extreme cases, such as advanced Alzheimer’s disease, all aspects of a person. Typically, people retain their personality and, in most cases, their ability to take responsibility for themselves.
In retrospect, early biological models of the mind seem impoverished and deterministic—for example, models that held that “levels” of a neurotransmitter such as serotonin in the brain were the principal influence on whether one was depressed or aggressive. Neuroscience is far beyond that now, working to integrate information coming “bottom-up” from genes and molecules and cells, with information flowing
“top-down” from interactions with the environment and experience to the internal workings of the mind and its neuronal circuits. Ultimately, however, the goal is not only human self-understanding. In knowing eventually precisely what goes wrong in what circuits and what synapses and with what chemical signals, the hope is to develop treatments with greater effectiveness and with fewer side effects. Indeed, as the following chapters indicate, the hope is for cures and ultimately for prevention. There is every reason to hope that as our science progresses, we will achieve those goals.
1 Special thanks to Steven E. Hyman, M.D., Director, National Institute of Mental Health, and Gerald D. Fischbach, M.D., Director, National Institute of Neurological Diseases and Stroke, for their contributions to this section.
Back to Top
Home | Contents | Previous | Next
|