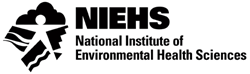
Environmental Factor, July 2008, National Institute of Environmental Health Sciences
Cline Explores Circuits in the Developing Brain
By Robin Arnette
August 2008
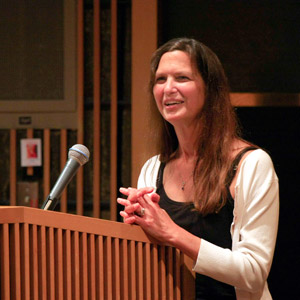
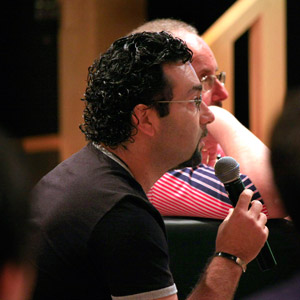
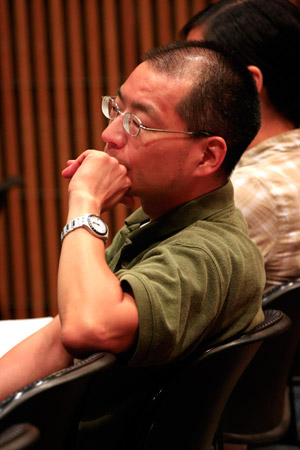
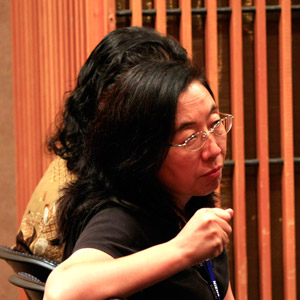
The latest NIEHS distinguished lecture in the 2007–2008 series featured Cold Spring Harbor Laboratory professor Hollis T. Cline, Ph.D., and was hosted by NIEHS Principal Investigator Serena Dudek, Ph.D. (http://www.niehs.nih.gov/research/atniehs/labs/ln/sdp/index.cfm) Cline presented "Mechanisms of Circuit Development: The Contribution of Synapse Elimination" on July 8 in Rodbell Auditorium.
Cline (http://www.cshl.edu/public/SCIENCE/cline.html) is interested in how connections develop within the human brain and how these connections are affected by experience in the relatively simple system present in Xenopus (frog) tadpoles. Work from several labs, including Cline’s, have shown that experience affects the frog’s visual system, which consists of the retina, optic nerve and optic tectun (OT), a region of the amphibian brain that is analogous to the superior colliculus in the human brain. Cline explained why this part of the brain was important. “The optic tectun and the superior colliculus are where different sensory modalities converge and regulate motor output. For instance, if you want to kick a soccer ball, you have to see the ball, know where the other players are, and then you can kick it.”
In Xenopus the optic nerve is a bundle of axons which sends signals from the retinal ganglion cells to OT cells through connections called synapses. The OT cells process the input and send output to the spinal chord. During frog development, the structure of the ganglion cell axons or axonal projections undergo large-scale arrangement. "The way they do that is by breaking synapses, migrating into a different part of the OT territory and re-establishing new synapses under the guidance of sensory experience," Cline said.
The axons of retinal ganglion cells, which constitute the pre-synaptic cells in this pathway, aren’t the only ones with the ability to move around in response to visual input. The postsynaptic cells in the OT extend processes toward the axons called dendrites. Cline discovered that dendrites have the same capacity for reorganization. Cline and colleagues used a technique called single cell electroporation to introduce green florescent protein (GFP) plasmids into single neurons of a tadpole. The whole organism was placed under a two-photon microscope and a staff member collected a Z-series of time-lapse photos through the neurons of the intact anesthetized animal. The photos revealed that the dendrites, called the dendritic arbor, looked like growing trees with many branches. Images taken at shorter intervals showed that the average lifetime of a branch was only 10 minutes.
Cline hypothesized that visual activity was stabilizing some of the branches so that they could contribute to the growth of the arbor. To test her supposition, she took images of a tadpole with a single tectal cell expressing GFP. She placed the animal in the dark for four hours and took another photo. Then, she placed the animal in a chamber that had light emitting diodes (LEDs) that flashed off and on for four hours. Cline took another micrograph of the tadpole and compared the three photos. She observed that the dendritic arbor experienced an increased growth rate during the period of visual stimulation.
Using electron microscopy, the team reconstructed an entire neuron and mapped the location of every synapse. The micrographs indicated that a branch could be extended, retracted or stable depending on the amount of synapses. "Branches that are actively extending have the highest synapse density, while those that are retracting have relatively few synapses," Cline stated. This means that many extra synapses are made on extending branches and they are retracted as the branches stabilize.
Cline’s work has shown that axons and dendrites use different synapse formation strategies when switching between extending and stable branches. Axons initiate new contacts with postsynaptic partners by sharing pre-existing synaptic contacts; dendrites add new branches, which are the primary site of synaptogenesis. Diseases or agents which affect branch addition, synapse formation or synapse elimination will affect circuit development and brain function.
"PCBs Are Topic..." - previous story
next story - "Laboratory of Structural Biology..."
August 2008 Cover Page