Abstract

Photo by: Tim McCabe, USDA-NRCS |
To minimize the impact of drought, soil needs to capture the rainwater that falls on it, store as much of that water as possible for future plant use, and allow for plant roots to penetrate and proliferate. These conditions can be achieved through management of organic matter, which can increase water storage by 16,000 gallons per acre foot for each 1% organic matter. Organic matter also increases the soil's ability to take in water during rainfall events, assuring that more water will be stored. Ground cover also increases the water infiltration rate while lowering soil water evaporation. When all these factors are taken together, the severity of drought and the need for irrigation are greatly reduced.
Table of Contents
Introduction
With severe drought an all-too-common occurrence, some farmers turn to irrigation for a solution. Irrigation may not be feasible or even desirable. Fortunately, there are management options that can increase the soil's ability to store water for plant use. Soil can be managed in ways that reduce the need for supplemental watering and increase the sustainability of the farm. This publication details some of the strategies for drought-proofing soil and the concepts that support them. Any worthwhile strategy for drought management optimizes the following factors:
- Capture of a high percentage of rainfall (infiltration)
- Maximum storage of water in the soil for later use (water holding capacity)
- Efficient recovery of stored water (plant rooting)
Several important soil factors affect water management—including soil texture, aggregation, organic matter content, and surface ground cover.
Back to top
Texture
Texture refers to the proportions of sand, silt, and clay present in a given soil. A sandy loam, for example, has much more sand and much less clay than does a clay loam. A loam soil is a more balanced blend of sand, silt, and clay. Most soils are some type of loam. Texture is an innate characteristic of the soil type. Unlike the other factors discussed here—aggregation, organic matter, and ground cover—texture cannot be changed through agronomic practice. By knowing the innate texture of the soil, however, the farmer can select and adjust practices that optimize moisture management.
Soil moisture-holding capacities corresponding to texture designations are found in Table 1. Notice that although the plant-available water is highest in the loam to clay-loam textures, the total water goes up with increasing clay content. This is because clay has more total pore space to hold water, but some of these pores are so small that the water is held too tightly for plants to extract. Sand has less total pore space to hold water, but most of the water it can hold is available to plants. Finally, water evaporation from sandy soils is faster than from clay soils. As any farmer knows, sandy soils dry out more quickly after a rain and plants growing on them show drought signs sooner compared to finer-textured soils. Consequently, it is wise to put drought-tolerant crops on the most drought-prone soils, and drought-sensitive crops on finer-textured soils.
Table 1. Soil texture's effects on soil moisture (1) |
Texture Designation |
Total Water Inches/foot |
Available Water Inches/foot |
Sand |
1.2 |
0.9 |
Sandy loam |
1.9 |
1.3 |
Fine sandy loam |
2.5 |
1.7 |
Loam |
3.2 |
2.0 |
Silt loam |
3.5 |
2.1 |
Sandy clay loam |
3.7 |
2.1 |
Clay loam |
3.8 |
2.0 |
Silty clay loam |
3.8 |
1.7 |
Clay |
3.9 |
1.5 |
Back to top
Aggregation
Soil aggregation refers to how the sand, silt, and clay come together to form larger granules. Good aggregation is apparent in a crumbly soil with water-stable granules that do not disintegrate easily. Well-aggregated soil has greater water entry at the surface, better aeration, and more water-holding capacity than poorly aggregated soil. (2) Plant roots occupy a larger volume of well-aggregated soil; better rooting increases the depth and area plants can reach for water. These are all positive attributes for drought resistance.

Figure 1. Effects of aggregation on water and air entry into the soil.
Derived from: Land Stewardship Project Monitoring Toolbox (3) |
Well-aggregated soil also resists surface crusting. The impact of raindrops causes crusting on poorly aggregated soil by disbursing clay particles on the soil surface, clogging the pores immediately beneath, sealing them as the soil dries. Subsequent rainfall is much more likely to run off than to flow into the soil. (Figure 1) In contrast, a well-aggregated soil resists crusting because the water-stable aggregates are less likely to break apart when a raindrop hits them. Take note, however, that any management practice that protects the soil from raindrop impact will decrease crusting and increase water flow into the soil. Mulches and cover crops serve this purpose well, as do no-till practices which allow the accumulation of surface residue.
A soil's texture and aggregation determine air and water circulation, erosion resistance, looseness, ease of tillage, and root penetration. However, while texture is an innate property of the native soil and does not change with agricultural activities, aggregation can be improved or destroyed readily through our choice and timing of farm practices.
Some practices that destroy or degrade soil aggregates are:
- Excessive tillage
- Tilling when the soil is too wet or too dry
- Using anhydrous ammonia, which speeds the decomposition of organic matter
- Excessive nitrogen fertilization
- Excessive sodium buildup from salty irrigation water or sodium-containing fertilizers
Aggregation is closely associated with biological activity and the level of organic matter in the soil. The gluey substances that bind components into aggregates are created largely by the various living organisms present in healthy soil. Therefore, aggregation is increased by practices that favor soil biota. Because the binding substances are themselves susceptible to microbial degradation, organic matter needs to be replenished to maintain aggregation. To conserve aggregates once they are formed, minimize the factors that degrade and destroy them.
The best-aggregated soils are those that have been in long-term grass production. (4) A grass sod extends a mass of fine roots throughout the topsoil, contributing to the physical processes that help form aggregates. Roots continually remove water from soil microsites, providing local wetting and drying effects that promote aggregation. Roots also produce food for soil microorganisms and earthworms, thus generating the compounds that bind the aggregates into water-stable units. Additionally, a perennial grass sod provides protection from raindrops and erosion while these processes are occurring.
This combination of factors creates optimal conditions for establishing a well-aggregated soil under a perennial cover. Conversely, cropping sequences that involve annual plants in extensive cultivation provide less vegetative cover and organic matter, and usually result in a rapid decline in soil aggregation and organic matter. No-till cropping requires less manipulation of the soil and retains a surface mulch; it is quite successful at promoting good aggregation on annually cropped soils.
Back to top
Organic Matter and Water-Holding Capacity
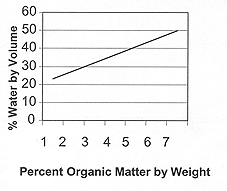
Figure 2. Available water content with increasing soil organic matter. (6) |

Figure 3. Effect of straw rate on water infiltration on a silt loam soil. (7) |
Soil holds water according to its texture, as shown in Table 1. However, the level of organic matter also determines how much water a soil can hold. Arkansas soil scientists report that for every 1% of organic matter content, the soil can hold 16,000 gallons of plant-available water per acre of soil down to one foot deep. (5) That is roughly 1.5 quarts of water per cubic foot of soil for each percent of organic matter. Figure 2 shows the relationship of organic matter to water-holding capacity.
Back to top
Ground Cover
The most apparent benefit of maintaining ground cover on soil is erosion resistance. However, ground cover is also associated with drought-proofing. This has been well demonstrated by a research team at Indiana using variable application of straw. Higher application resulted in higher water infiltration rates, up to 2.5 tons of straw per acre. (Figure 3)
Table 2. Water entry into the soil after 1 hour (2) |
Manure Rate (Tons/acre) |
Inches of water |
0 |
1.2 |
8 |
1.9 |
16 |
2.7 |
Surface cover also reduces water evaporation from soil. In a Kentucky study, surface evaporation was five times less under no-till (which leaves a surface mulch) than with conventional tillage over the May to September season. (Table 3) Because less water was lost to evaporation, more water was available for plants.
Table 3. Water evaporation and transpiration* from tillage types over a 5-month growing season (8) |
Tillage type |
Evaporation
mm of water |
Transpiration*
mm of water |
No-till |
41 |
307 |
Conventional Till |
191 |
242 |
* Transpiration is the release of water vapor by plants. |
Tillage systems and equipment have enormous impacts on water infiltration, storage, and plant efficiency. These include mechanical stress on soil aggregates, effects on soil microorganisms, and the tendency to create hardpans. Of most importance to drought-proofing, however, is the extent to which a surface cover is maintained.
Table 4. Tillage effects on water infiltration and ground cover (2) |
|
Water Infiltration
mm/minute |
Ground Cover
Percent |
No-till |
2.7 |
48 |
Chisel Plow |
1.3 |
27 |
Moldboard Plow |
0.8 |
12 |
Table 4 shows three different tillage methods and how they affect water entry into the soil. Notice the direct relationship between tillage type, ground cover, and water infiltration. No-till has more than three times the water infiltration of moldboard-plowed soil. Additionally, the no-till field would have higher aggregation from the organic matter decomposing on-site. Table 5 shows increases in water infiltration in a dry soil between no-till and conventional tillage systems; water continues to infiltrate well after the soil becomes wet. An often-ignored factor in soil water infiltration is the contribution made by earthworm channels. In soils with high earthworm populations, 30 to 50 nearly pencil-sized vertical tunnels per square yard are common. (10) Gently sloping land under a mid-summer corn canopy can absorb a 4-inch rainfall in 2 hours with virtually no runoff when abundant earthworm populations are present. (10)
Table 5. Effects of tillage on water infiltration on a silt loam soil (9) |
Tillage History |
Dry Soil
mm water/hour |
Wet soil
mm water/hour |
No-till |
43 |
22 |
Conventional till |
31 |
10 |
Gantzer and Blake (11) examined soil channels created by earthworms and plant roots in a clay loam soil in Minnesota. At the 3- to 6-inch soil depth, the abundance of channels from roots and worms was approximately three times higher under no-till than where the soil was fall plowed. (Table 6) Of course with more channels, the rate of water infiltration is higher, resulting in more water capture from each rainfall.
Table 6. Soil earthworm and plant root channels at the 3- to 6-inch depth during two seasons, under two tillage regimes (11) |
Season |
No-till
channels/m² |
Fall plow
channels/m² |
Spring |
1317 |
420 |
Fall |
635 |
216 |
The data in Table 7 point out that increased ground cover enhances water storage as well as infiltration. The surface mulch typical of no-till fields acts as a protective skin to the soil, reducing the impact of raindrops, buffering the soil from temperature extremes, and reducing water evaporation.
Tillage also affects plant rooting, and thereby directly affects the efficiency of water withdrawal by crop plants. Because it prunes roots and dries the soil surface, row-crop cultivation discourages shallow rooting. No-till, in contrast, encourages an abundance of roots near the soil surface. Since water infiltration and storage are higher under no-till, plant roots find the water and nutrients they need closer to the surface.
Table 7. Effect of tillage on ground cover and water storage (12) |
Tillage System |
% Ground Cover |
% Water Stored |
No-till |
78 |
130 |
Stubble mulch |
38 |
123 |
Bare ground |
5 |
100 |
In some no-till situations, total root volume is less than in plowed soils. One study showed 34% fewer corn roots at the 2-foot depth under no-till. (13) A well-structured Indiana soil showed similar total corn root weights under no-till but more of the roots were in the top 3 inches of soil. (14) Deep tillage encourages deep rooting; subsoiling can increase rooting depth and impart increased drought protection. Before subsoiling, determine whether it is necessary. Push a sharpened steel probe in the ground to test for compaction. If you are going to be planting on the same rows year after year (as with ridge-till), probe on top of the rows.
Additionally, you can dig a 2-foot-deep hole and run a knife blade through the sidewall of the hole to check for resistance. Where the knife blade stops is where the hardpan is. When adjusting the depth of the subsoiler shank, you will want to run it just under the compacted layer. For example, if the bottom of the layer is 9 inches deep, then run the shank at 10 or 11 inches, not 12 or 13. Running as shallow as necessary will reduce draft requirements and cost. Subsoiling shanks can also be run in-row, leaving the surface largely undisturbed. The in-row surface layer must be firmed up to prevent the seed from falling deep into the subsoil cracks, however. This is typically done with attachments on the planter.
No-till and reduced-tillage systems benefit soil. The advantages of a no-till system include superior soil conservation, moisture conservation, reduced water runoff, long-term buildup of organic matter, and increased water infiltration. A soil managed without tillage relies on soil organisms to take over the job of plant-residue incorporation formerly done by tillage. On the down side, no-till can foster a reliance on herbicides to control weeds and can lead to soil compaction from the traffic of heavy equipment. Pioneering development work on low-chemical no-till farming is proceeding at several research stations in the eastern U.S. and on at least one farm.
Back to top
Farmer Profiles
In reviewing many articles for this publication, I saw numerous farmer testimonials for increased drought tolerance and higher water capacity from no-till. Here are three examples:
-
Georgia coastal plain farmer Lamar Black applied irrigation water at a rate of 1.5 inches per hour on his 1993 corn crop that had been converted to no-till. The year before, that same field would only take in ½ inch of irrigation water per hour—a higher rate produced runoff. (15)
-
David Iles of North Carolina says his silage yields averaged 12 to 15 tons per acre with adequate rainfall and 4 to 5 tons without it before switching to no-till. After no-till, he makes 20 tons per acre with adequate rainfall and 10 tons without enough rainfall. David says that water is his limiting factor. If he gets rain he will make top yields, since switching to no-till. He realizes that organic matter is the engine that drives his system and provides food for earthworms and microorganisms. David Iles built his soil by fallowing out 20 to 25 acres of his 380-acre farm each year. On these fallow acres he spreads manure and then sows crops that are not harvested but grown as a green manure for their organic matter. Even weeds are not clipped but left for their organic matter. David loves his earthworms and says they are the best employees he has. "They work all the time and eat dirt for a living." (16)
-
Pennsylvania farmer Steve Groff has been farming no-till with minimal herbicides and extensive use of cover crops for 15 years in Lancaster County, Pennsylvania. In the spring he rolls the cover crops down using a 10-foot rolling stalk chopper, which kills the rye or vetch and creates a nice no-till mulch into which he plants a variety of vegetables and grain crops. After several years of no-till production, his soils are mellow and easy to plant into. Steve farms 175 acres of vegetables, alfalfa, and grain crops on his Cedar Meadow Farm. He has a video showing how he manages his cover crops and plants into them:
No-till Vegetables by Steve Groff. 1997. 35 minutes.
This video leads the viewer from selection of the proper cover crop mix to plant into, to how to control cover crops with little or no herbicide using mechanical cover-crop-kill methods, to no-till transplanting of vegetables. Includes comments from leading researchers in no-till vegetable production. Available for $21.95 + $3.00 shipping from:
Cedar Meadow Farm
679 Hilldale Road
Holtwood, PA 17532
717-284-5152
Summary
High aggregation, abundant surface crop residue, and a biologically active soil are keys to drought-proofing a soil. All these qualities are advanced by reduced-tillage systems. In short, maintaining high residue and adding organic matter while minimizing or eliminating tillage promotes maximum water conservation.
Back to top
References
-
Rahn, J. J. 1979. Making the Weather Work for You. A Practical Guide for Gardener and Farmer. Garden Way Publishing, Charlotte, Vermont. 204 p.
-
Boyle, M., W.T. Frankenberger, Jr., and L.H. Stolzy. 1989. The influence of organic matter on soil aggregation and water infiltration. Journal of Production Agriculture.
Vol. 2. p. 209-299.
-
Land Stewardship Project. 1998. The Monitoring Toolbox. White Bear Lake, MN. Page number unknown.
-
Allison, F.E. 1968. Soil aggregation-some facts and fallacies as seen by a microbiologist. Soil Science. Vol. 106, No. 2. p. 136-143.
-
Scott, H.D., L.S. Wood, and W.M. Miley. 1986. Long-term effects of tillage on the retention and transport of soil water. Arkansas Water Resources Research Center. Publication Number 125. 39 p.
-
Hudson, B. E. 1994. Soil organic matter and available water capacity.
Journal of Soil and Water Conservation. Vol. 49, No. 2. p. 189-194.
-
Mannering, J.V., and L.D. Meyer. 1963. The effects of various rates of surface mulch on infiltration and erosion. Soil Science Society of America Proceedings.
Vol. 27. p. 84-86.
-
Phillips, R.E. 1980. Soil Moisture. In: R.E. Phillips, G.W. Thomas, and R.L. Blevens (eds.) No Tillage Research: Research Reports and Reviews, University of Kentucky, College of Agriculture and Agriculture Experiment Station, Lexington. p. 23-42.
-
McGregor, K.C., C.K. Mutchler, and R.F. Cullum. 1991. Soil erosion effects on soybean yield. Paper N. 912626. American Society of Agricultural Engineers,
St. Joseph, MO.
-
Staff. 1993. Land's best friend. In: Proceedings: National No-tillage Conference, 1993, Cedar Falls, IA. p. 317-320. [Reprinted from LandOwner.]
-
Gantzer, C.J., and G.R. Blake. 1978. Physical characteristics of Le Sueur clay loam soil following no-till and conventional tillage. Agronomy Journal. Vol. 70. p. 853-857.
-
Peterson, G.A., and C.R. Fenster. 1982. No-till in the great plains.
Crops and Soils Magazine. Vol. 43. p. 7-9.
-
Navas, A.J. 1969. The effect of several tillage systems on some soil physical properties and on corn growth. MS Thesis, Purdue University. August, Catalog number 22652, West Lafayette, IN.
-
Cruz, J. C. 1982. Effect of crop rotation and tillage systems on some soil physical properties, root distribution, and crop production. Ph.D. thesis, Purdue, University,
West Lafayette, IN.
-
LaRose, J., and J. Dirnberger. 1996. The little pluses add up. National Conservation Tillage Digest. Fall. p. 5-7.
-
Dirnburger, J.M., and M. LaRose. 1997. No-till saves dairy farm by healing the harm that tillage has done. National Conservation Tillage Digest. Summer. p. 5-8.
Drought Resistant Soil
By Preston Sullivan
NCAT Agriculture Specialist
Tiffany Nitschke, HTML Production
IP169
Slot 127
Back to top |