Monitoring Volcano Ground Deformation
Changes at the Surface Tell us about the Subsurface
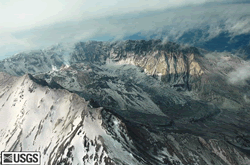
Changes to the surface of a volcano (volcano deformation) can provide clues about what is happening deep below the surface. Most volcano deformation can only be detected and measured with precise surveying techniques. The Volcano Hazards Program has installed networks of sensitive deformation instruments around volcanoes to monitor changes over time. These instruments, along with satellite-based technologies help us to better understand the volcanoes we watch and allow us to provide eruption warnings.
Global Positioning System (GPS)
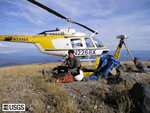
The Global Positioning system consists of a constellation of 24 satellites. Each satellite orbits Earth twice a day at an altitude of about 20,000 km and continuously transmits information on specific radio frequencies to ground-based receivers. GPS was developed by U.S. Department of Defense as a worldwide navigation system and has been adopted by civilians for many other uses, including surveying, mapping and scientific applications. Relatively inexpensive GPS receivers like those used by pilots, boaters and outdoor enthusiasts can determine its position on the Earth‘s surface to within a few tens of meters. With more sophisticated receivers and data-analysis techniques, as shown on Mount St. Helens, we can determine receiver positions to less than a centimeter.
How does GPS work?
GPS satellites continuously transmit an estimate of the satellite’s position, a digital code, and the precise time of the signal. GPS receivers measure distance using the travel time of the radio signals. Distance is calculated by multiplying the time it takes the radio signals to reach the receiver times the speed at which the signals travel - approximately 186,000 miles/second (the speed of light). Knowing where the satellites are located when they transmit their signals, the receiver can calculate its position on Earth or in the air. The key is that receivers must simultaneously receive the signals from at least 4 satellites, in part because the clocks in the receivers aren‘t as accurate as the atomic clocks in the satellites. If the clocks in a receiver and satellite were out of sync by 1/1000th of a second, the distance measurement could be off by 186 miles! The fourth measurement essentially enables the receiver to correct its internal clock.
For an online overview of how GPS works, see Trimble Navigation's tutorial.
Using GPS Networks to Gain More Accurate Data
The current constellation of satellites provides the GPS user with 5 to 8 satellites in view from anywhere on Earth, if one has an unobstructed view of the sky in all directions. With this much information, a GPS receiver can very quickly determine its position to within a matter of meters. On volcanoes, however, an accuracy of a few centimeters or less is extremely important for detecting the build up of stress and pressure caused by magma rising toward the ground surface. To obtain this kind of accuracy in our measurements, we need to take other factors into account, including the variation in the speed of the signal transmitted from the satellite as it travels through the atmosphere and the uncertainty in the position of the satellite.
A common way of eliminating these potential errors is to set up GPS receivers over several volcano benchmarks at the same time so that we can simultaneously collect data from the same satellites. Since most of the error associated with the delay of the signal through the atmosphere and the location of the satellites becomes the same for all sites, we can determine their positions relative to one another to less than a centimeter. For the greatest accuracy, we collect GPS data for 8 to 24 hours and then calculate the position of the benchmark utilizing more precise satellite locations and modeling the atmospheric delay.
Tiltmeters
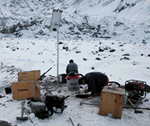
Scientists install a new tiltmeter site north of the west arm of the Crater Glacier. View is looking south with the snout of the west arm of the Crater Glacier the background. Measuring tiny changes in the slope angle or “tilt” of the ground at a volcano is one of the oldest methods for monitoring deformation caused by moving magma. When magma forces the ground up, the slope of adjacent areas will usually tilt away from the center of uplift by only a fraction of a degree. Conversely, if the ground subsides as a consequence of magma moving below, the slope of adjacent areas will tilt toward the center of subsidence. We use electronic tiltmeters for continuously recording such ground tilts on volcanoes, and they have become the most widely used instrument for measuring volcano ground deformation in real time.
What is an electronic tiltmeter?
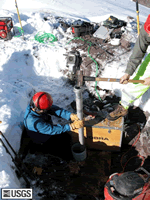
Like a carpenter's level, an electronic tiltmeter uses a small container filled with a conducting fluid and a “bubble” to measure a change in slope. Electrodes placed in the fluid and into the bubble determine the bubble's position--as the bubble moves, voltage output from the electrode changes in a way that correlates to the amount of tilt that caused the bubble to move.
Close-up view of a tiltmeter being installed at Soufriere Hills Volcano on the Caribbean Island of Montserrat, 1995.
How sensitive are tiltmeters?
Tiltmeters measure the amount of tilt in microradians, which is the angle turned by raising one end of a beam one kilometer long the width of a dime (equivalent to 0.00006 degree!). Originally designed as part of the guidance and control system for military missiles, a variety of electronic tiltmeters are now available for volcano monitoring, each with different resolutions and ranges. For example, we use tiltmeters with ranges of between 100 and 10,000 microradians depending on the volcano and expected degree of tilt.
Selected case histories of tilt at volcanoes (from the old site)
- A Remarkable Pattern of Tilt at Kilauea Volcano, Hawai`i
- Eruption prediction aided by tiltmeters at Mount St. Helens
References
D. Dzurisin, 1992, Electronic tiltmeters for volcano monitoring: lessons from Mount St. Helens, in Ewert, J.W., and Swanson, D.A. (eds), Monitoring volcanoes: techniques and strategies used by the staff of the Cascades Volcano Observatory, 1980-90, U.S. Geological Survey Bulletin 1966, p. 125-134.
Murray, T.L., Ewert, J.W., Lockhart, A.B., and LaHusen, R.G., 1996, The integrated mobile volcano-monitoring system used by the Volcano Disaster Assistance Program (VDAP), in Scarpa, R. and Tilling, R.I. (eds), Monitoring and mitigation of volcano hazards, Springer-Verlag Berlin, p. 315-362.
Interferometric Synthetic Aperture Radar (InSAR)
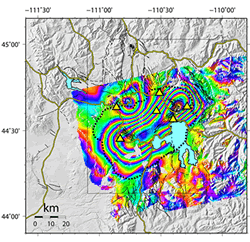
InSAR, such as the image shown here from Yellowstone, provides infrequent (annual to semi-annual) but highly detailed images of the amount of movement of the ground relative to a satellite. The technique is very useful for monitoring ground movements or ground deformation at volcanoes because detailed data can be collected for a relatively large area from space. By aiming a pulse of radar energy at the ground and then recording information about the return signal, such as the time traveled by the radar waves, InSAR quickly provides data for an entire area. By returning to the same area at different times, we can gain information about relative ground movements over time.
The InSAR data are processed into images, called interferograms. Ground movement, either up or down, is shown by the changes in color on the processed images. InSAR data can be combined to produce very high-resolution images that compare changes from one year to the next or multiple passes can be combined to show changes over a few years of time.
For more information, please see our VHP InSAR Research Group pages.
Electronic Distance Meter (EDM)
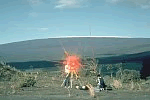
Measuring the distance between benchmarks placed on a volcano tens to thousands of meters apart can sometimes pinpoint where and when magma is rising toward the surface. Rising magma will sometimes push overlying rocks upward or shove them aside. In either case, one part of the volcano may actually move horizontally relative to another part from as little as a few millimeters to as much as several tens of meters. The challenge in measuring such changes with an electronic distance meter is putting benchmarks in the right places and making frequent measurements between pairs of benchmarks.
What is an electronic distance meter?
An electronic distance meter is an instrument that both sends and receives an electromagnetic signal. Depending on the distance between the EDM and reflector, the wavelength of the returned signal will be out of phase with the transmitted signal. The instrument compares the phase of the transmitted and received signals and measures the phase difference electronically. There is a wide range of EDM capabilities in range and precision, but for volcano monitoring purposes, short-range (less than 10 km) to medium-range (less than 50 km) EDM's are typically used. Short-range EDM's transmit and receive the near visible infrared part of the electromagnetic spectrum for measuring distances with an accuracy of about 5 mm.