Stem cells are immature body cells that act like "starter dough" because they can make identical copies of themselves. This keeps a constant supply of "starter" cells ready to mature within several distinct tissue layers--internal, middle, or external--in response to the body's needs. Stem cells mature within these layers, replacing aging or damaged cells in their respective body tissues. Once they mature, stem cells lose the ability to duplicate themselves.
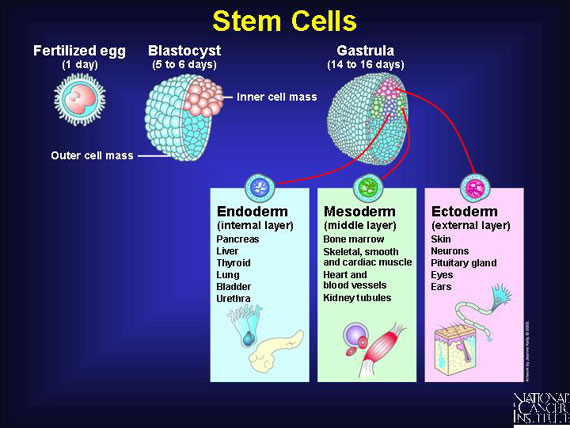
Blood stem cells, known as hematopoietic stem cells, reside primarily in marrow, the spongy interior of bones. These "starter" cells resupply three types of blood cells: erythrocytes, commonly known as red blood cells; platelets, also called the blood-clotting cells; and leukocytes, the white blood cells of the immune system.
When the body needs to replace red blood cells, platelets for blood clotting, or immune cells, stem cells located in the bone marrow mature in a process called hematopoiesis. Hematopoiesis goes on constantly in the human body, but certain conditions call for increased activity. For example, when a person moves to a high altitude or attempts to recover from serious bleeding, increased numbers of stem cells must move from the bone marrow into the bloodstream.
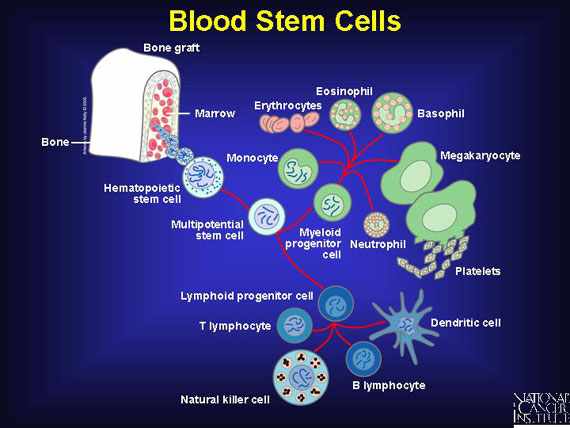
Immune cells--also called white blood cells or lymphocytes--leave the bone marrow while still immature, and they migrate through the bloodstream on their way to the thymus and other lymphoid organs. During their journey, they mature into specialized T or B cells of the immune system. Other blood components, like red blood cells, completely mature in the bone marrow before being released into the blood.
In general, at any given moment, there is one blood stem cell circulating in a person's bloodstream for every 100 present in bone marrow. Although it is not precisely understood how the body signals for more blood stem cells to move out of the marrow, scientists are studying some factors that seem to increase the rate of release.
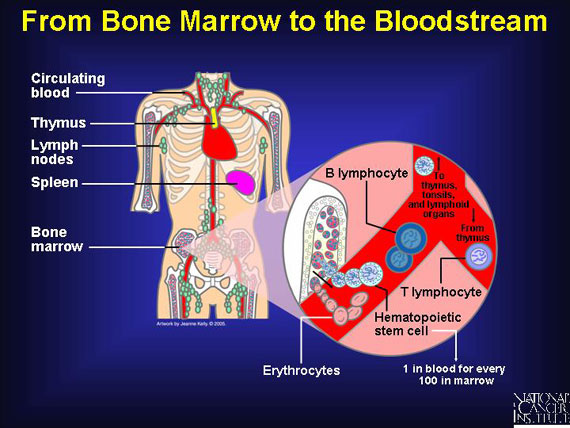
In patients with blood cancers such as myeloma, leukemia, or lymphoma, the chemotherapy and radiation that kill malignant cells also kill healthy marrow and its resident blood stem cells. Whether the cancer begins in the marrow itself or in lymphoid or other organs, anticancer treatment can deplete much of the body's reserve of blood-forming stem cells. Even patients with noncancerous blood diseases can develop a shortage of blood stem cells, as occurs in life-threatening anemias or immune disorders. In all these cases, a blood stem cell transplant can restore normal hematopoiesis.
Hematopoietic or blood stem cell transplants are also called bone marrow transplants or peripheral blood stem cell transplants, depending upon the location of stem cell collection.
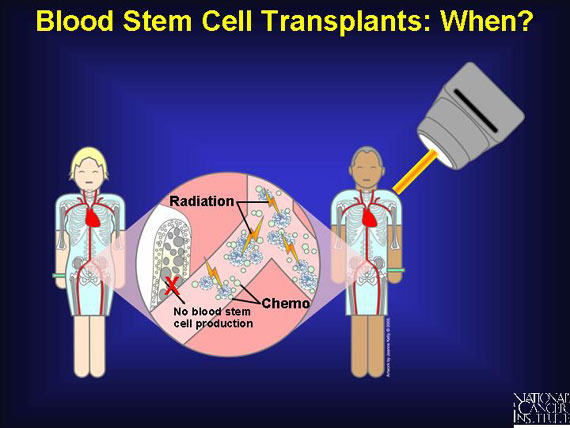
To minimize any damage to blood stem cells from cancer treatment, these stem cells from the bone marrow are removed and preserved before patients receive chemotherapy. The stem cells are then re-infused into the patient after chemotherapy where they migrate to the bone marrow and begin producing new blood cells. Patients with multiple myeloma as well as non-Hodgkin's and Hodgkin's lymphoma may be offered this approach as part of their treatment.
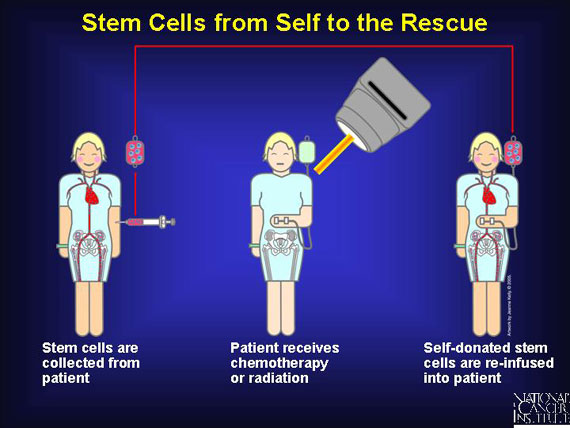
Once collected, stem cells are usually infused immediately into the patient, where they migrate to the bone marrow and "settle in" or engraft. Once there, stem cells can repopulate the bloodstream with normal red blood cells and immune cells that "rescue" the patient. For some cancer types, this feat is curative.
Alternatively, stem cells are harvested from a donor source and "cryopreserved," which means they are frozen in liquid nitrogen. At some later time, they are thawed and infused into a patient.
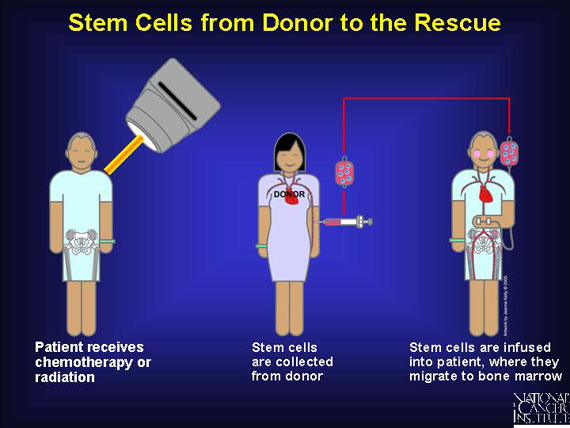
The success of a blood stem cell transplant relies upon the interactions of markers on the surface of all body cells, including the immune cells of both the patient and the donor. Normally, all cells within the patient's body coexist peacefully in a state known as self-tolerance because all bear the same "self" marker proteins. These proteins are also called antigens because, should they be introduced into a new environment, they are capable of stimulating a powerful immune reaction. This is what occurs when a blood stem cell preparation with "non-self" antigens is transplanted into a patient whose "self" antigens are very different.
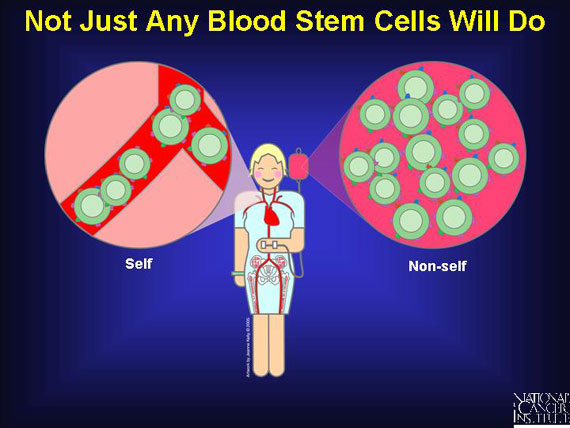
 |
Donated blood stem cell transplants bring with them their own distinctive "self" antigens. The immune system of the patient, who is called a host, senses that these antigens are "unmatched" or "non-self." This prompts the patient's immune cells to attack the donated (transplanted) cells, which are called a graft, and this assault can lead to the patient's rejection of the transplant ("host vs. graft").
More often, though, some mature donor immune cells that are mixed in among the transplanted blood stem cells recognize the antigens on the patient's body cells (host) as "non-self." This causes the transplant to attack the patient's tissues and organs. The result is "graft vs. host" disease, which can be very serious.
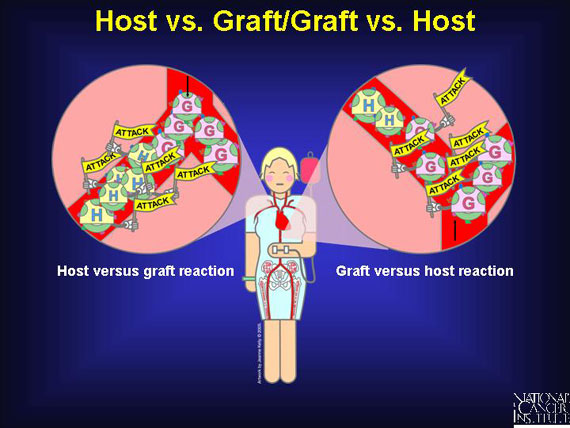
Success in transplanting blood stem cells depends on finding a compatible match of the antigens on donor's cells and tissues with those on the patient's. In most cases, this means finding sets that are slightly different, but not too different. Donors need not be related to the patient, but they must have a reasonable amount of compatibility. A blood test known as "tissue typing" is used to find good matches.
When patients donate their own stem cells (autologous), "self" antigens meet "self" antigens and there is enough similarity to lessen the risk of immune cell warfare. The same advantages are associated with syngeneic (from identical twin) transplants, although few patients have identical twins.
In most cases, the transplant is allogeneic, meaning the blood-forming stem cells come from a "non-self" donor. These transplants will contain antigens that are somewhat like--but not identical to--those of the patient.
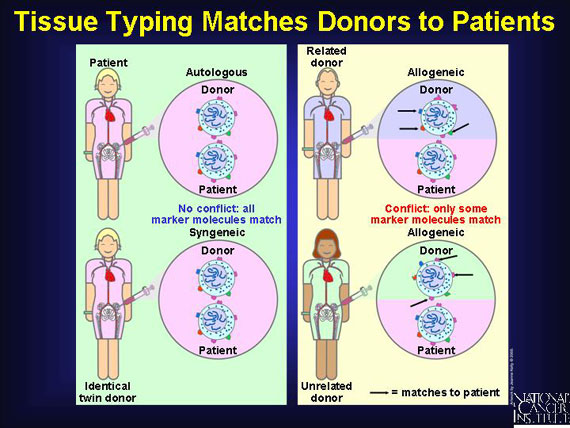
The scientific name for "self" antigens is major histocompatibility complex (MHC) proteins, and each person bears a unique set. To make matters even more confusing, when these MHC proteins mark the surface of immune cells called leukocytes, the antigens are dubbed human leukocyte antigens (HLAs). Since leukocytes are the cells used for tissue typing, HLAs are what clinicians use to find reasonable matches for their patients. Whether called MHC proteins or HLAs, all are the same thing--"self" antigens. Technicians remove human leukocytes from the blood of potential donors and determine what HLA markers are present.
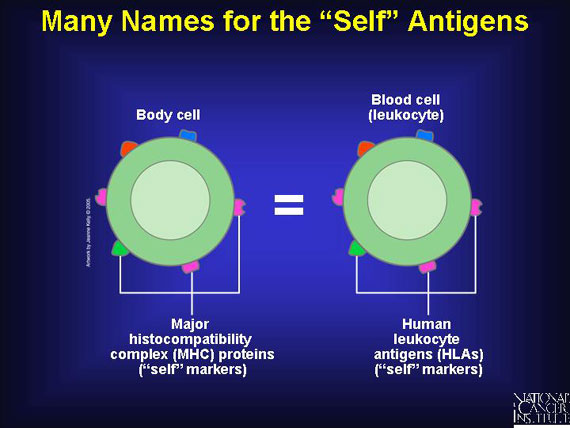
 |
Genes for "self" antigens are passed on from one generation to the next in a clump called a haplotype. A haplotype is a combination of genes--HLA alleles, for example--that are located closely together on the same chromosome and tend to be inherited together as a package deal. Alleles are genes that come in many varieties, all of which function reasonably well at making the related protein.
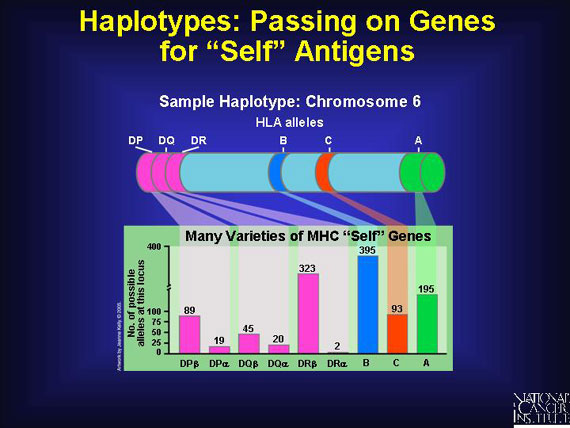
Six important genes for human leukocyte antigens are contributed by each parent. So each person inherits a double set of six major genes that produce six major corresponding antigens, HLA-A, -B, -C, -DP, -DQ, and -DR. Since as many as 20 varieties exist for each of these HLA-producing genes in different individuals, the total possible human leukocyte antigens that can mark "self" and trigger an immune response number about 10,000!
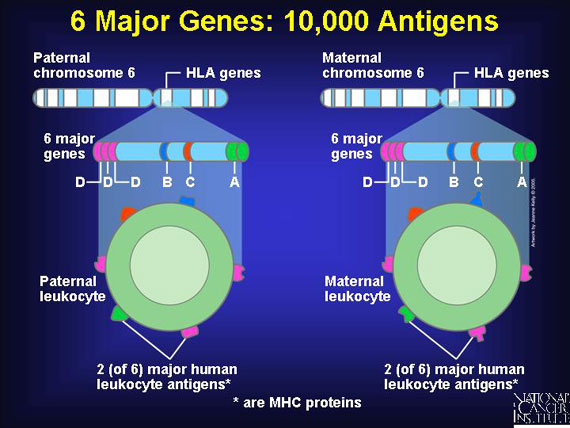
Some donor "self" antigens are more antigenic than others, which means they are better at triggering an unwanted immune reaction in the transplant patient. By studying successful cases of blood stem cell transplantations, doctors have identified the three most important antigens to match when choosing "non-self" donors for a transplant. Matching these helps to minimize the chances of graft vs. host or host vs. graft attacks. The antigens are HLA-A, HLA-B, and HLA-DR.
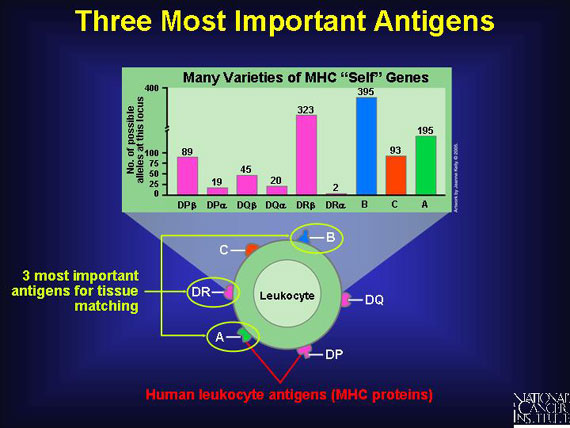
An ideal 6:6 antigen match, known as a "clinical match," means that both sets of the three "most important" inherited human leukocyte antigens in the donor match perfectly with those on the body (and immune) cells in the patient. Below, only Child E would serve as a 6:6 clinically matched donor for Child A; one sibling is a total mismatch; the other siblings and both parents would be haplo-identical because only one of their two inherited haplotypes matches Child A.
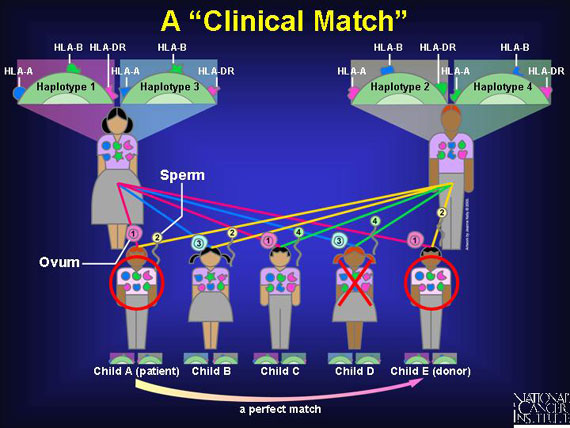
Because certain haplotypes are inherited among siblings more frequently than expected merely by chance, there is a 25-30 percent probability of finding a 6:6 match within a family. If one antigen is mismatched, it is a 5:6 match, which clinicians will accept because it increases the odds of finding a donor. When doctors accept a 4:6 match, the likelihood of finding a donor increases even more.
As the number of HLA antigen matches decreases among donors and recipients, the chances of finding a donor increase:
Antigen match Percent chance of finding a donor:
6-antigen match 25-30%
5-antigen match 40-50%
4-antigen match 50-60%
3-antigen match >90%
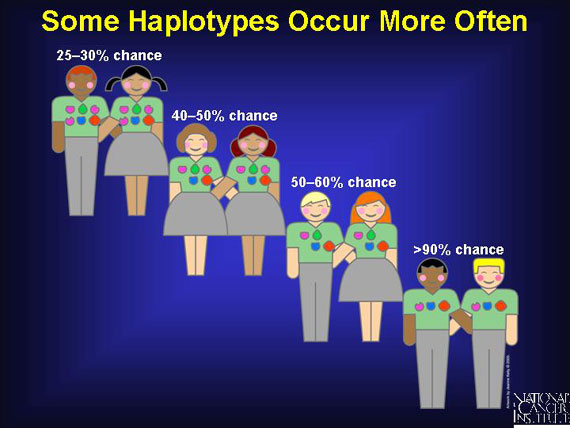
More than 90 percent of patients can find a haplo-identical donor if they are willing to accept a 3-antigen match. Sometimes this is medically necessary, as when severely ill patients cannot wait the several months it may take to find a 4-, 5-, or 6-antigen matched donor. Patients accepting a 3-antigen haplo-identical match also accept a serious risk of suffering an immune cell attack on their tissues by the transplant (graft vs. host disease), or of having their body reject the transplant (host vs. graft).

 |
Haplo-identical transplants may provide a possible benefit. They may enable an attack on cancer to occur. A graft vs. tumor or graft vs. leukemia effect occurs when a donor's mature immune cells come along with the stem cells in the transplant, and these immune cells recognize and attack as "foreign" the cancer cells found in the patient's body. This phenomenon helps prevent relapse and, in some cancers, can even be curative. To boost this graft vs. tumor effect, patients are sometimes given an infusion of a donor's haplo-identical immune cells (white blood cells) along with the stem cell transplant.
A risk with haplo-identical transplants is that of enabling an attack on the patient's tissues. This can happen when a donor's mature immune cells, called T cells, contaminate the stem cell graft. To minimize this risk, researchers sometimes deplete all T cells from the transplant, leaving the graft with stem cells only. While this can minimize the chance of graft vs. host disease, it also reduces the transplant's ability to attack any loitering cancer cells. An alternative option is for the doctor to prescribe steroid medications for the patient pre- and post-transplant. This helps to prevent graft vs. host disease, yet leaves T cells available for graft vs. tumor action.
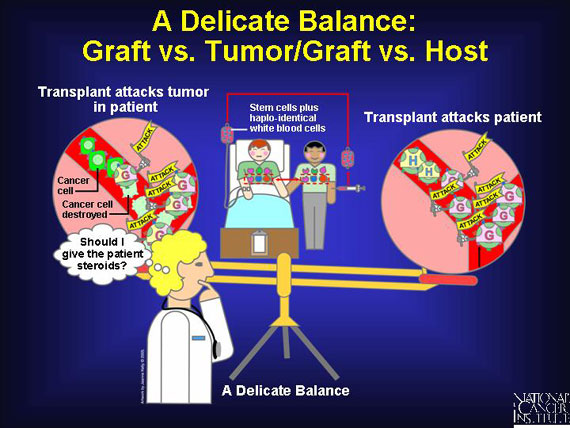
 |
An alternative to a haplo-identical transplant between related individuals is a matched transplant between unrelated individuals. For Japanese patients, because their island geography increases the likelihood of marriage within a limited gene pool, the chances of finding a 6:6 clinical match are better than for patients in larger gene pools. Of course, much depends upon getting enough donors. Like North American Caucasians, African American and Asian patients are part of a large gene pool, but they are under-represented in the donor pool and only have a 50 percent probability of finding a 6:6 clinical match.
Among different ethnic groups, the probability of finding a 6:6 match is, from the highest to lowest, Japanese (99%), North American Caucasian (93%), African American and Asian (50%).
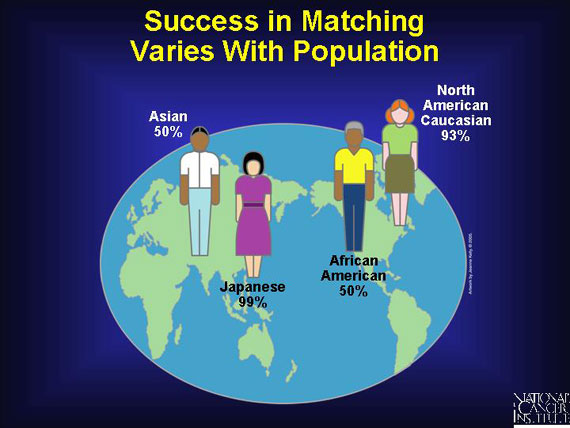
Before receiving allogeneic transplants--the most common type--patients with blood cancers must undergo a conditioning regimen of high-dose chemotherapy or radiation to kill any resident cancer cells, suppress the patient's immune system, and leave a disease-free environment into which healthy new blood stem cells can be infused. Known as myeloablation, the regimen prepares the patient to accept a "non-self" graft without triggering a severe attack on the patient by the transplant (graft vs. host disease).
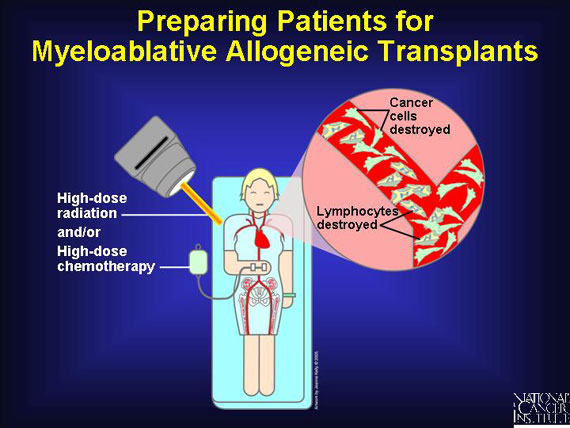
 |
Because many patients are unable to withstand myeloablation, clinical researchers are studying the feasibility of reduced-intensity nonmyeloablative regimens. Before infusing donated blood-forming stem cells, the patient receives low-dose or standard-dose chemotherapy and/or radiation therapy. Afterward, the patient is given immunosuppressant steroid drugs to help prevent the body from rejecting the transplant. Sometimes the patient also receives an infusion of donated immune cells (white blood cells from the same donor who supplied stem cells) to enhance the graft's attack on the patient's cancer (graft vs. tumor benefit).
This approach is new, so clinical researchers do not have extensive data on its effectiveness. Graft versus host disease sometimes occurs. So far, rates of graft vs. host disease using this approach are similar to those after high-dose conditioning regimens, but the onset is often delayed by weeks and months. Insurance companies and Medicare administrators, who view reduced-intensity transplants as experimental, often do not cover them.
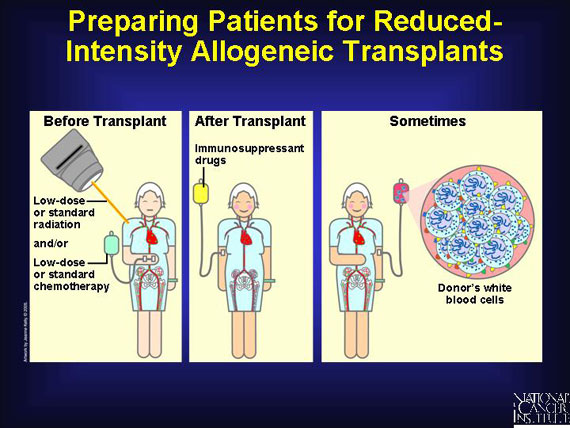
Blood stem cells are extracted most often from the peripheral blood of donors and occasionally from their bone marrow. Because there are significantly fewer stem cells in peripheral blood than in bone marrow, doctors prepare peripheral blood donors by injecting them with a series of growth factors to move blood stem cells from their marrow into their bloodstream. This increases the blood stem cells concentration 10- to 100-fold in the blood.
If stem cells are harvested from bone marrow, the donor is placed under general anesthesia. In a procedure that takes about an hour, marrow is removed through a large needle inserted into the donor's pelvic bones, and it is processed to remove blood and bone fragments. After the collection is completed, the donor may be given a transfusion of his or her own red blood cells from units of blood that were self-donated a week earlier.
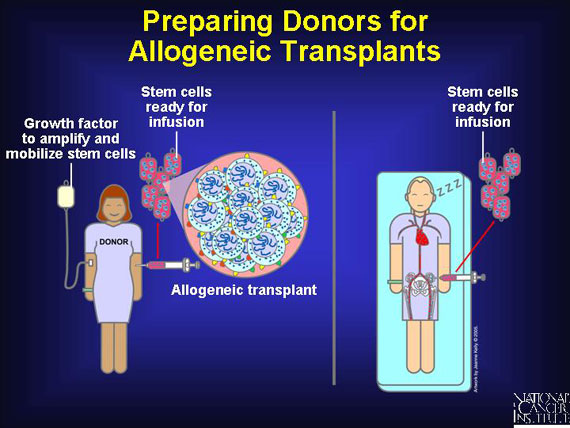
Nurses harvest blood stem cells from a donor's peripheral blood using a process called apheresis. This process involves removal of whole blood from the donor. As blood is drawn from the donor, circulating stem cells (and sometimes mature immune cells) are extracted. A centrifuge-type machine then separates the components. The blood itself, minus the stem cells, is returned to the donor. Apheresis requires no anesthesia but can take several hours.
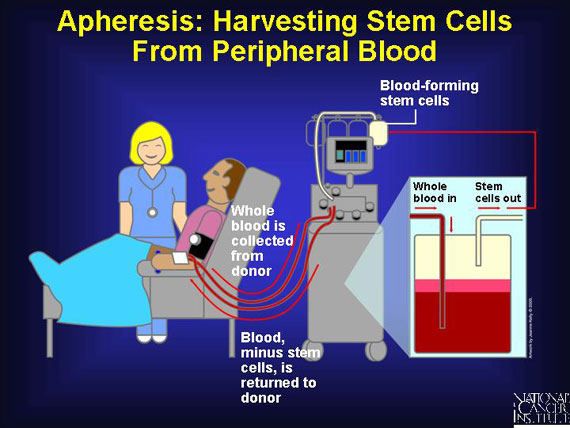
Like allogeneic donors, patients who donate their own peripheral blood for an autologous stem cell transplant, as well as identical twins making a syngeneic donation, receive injections of growth factor prior to collection by apheresis. This amplifies the number of blood stem cells in the bloodstream. Almost all autologous/syngeneic transplants are now taken from peripheral blood.
Of course, the patient will also receive high-dose chemotherapy before his self-donated stem cells are reinfused. Some clinical trials even repeat the procedure twice for these autologous transplants, so high-dose chemotherapy is followed by a self-donated transplant two times. This back-to-back clinical protocol is called a tandem transplant.
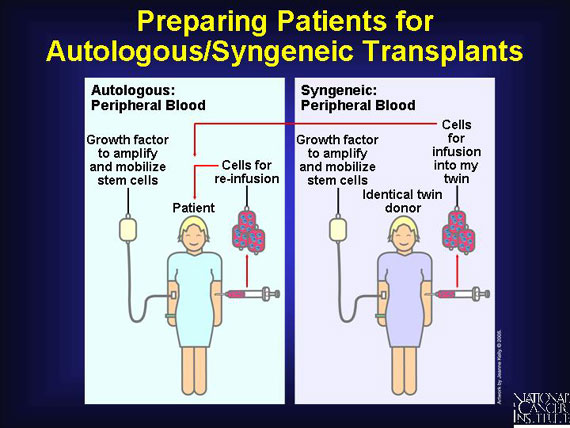
The human body's most primitive stem cells form in the fetal yolk sac and move to the fetal liver before entering the baby's bone marrow during the third trimester of pregnancy. When a baby is born, the placenta and umbilical cord still contain a rich supply of immature blood stem cells in the very process of migrating. These stem cells are capable of rebuilding all three types of blood cells in the body (red blood cells, immune cells, and platelets). First used in 1989 to help children with leukemia, cord-blood transplants have since proven themselves effective for adults too.
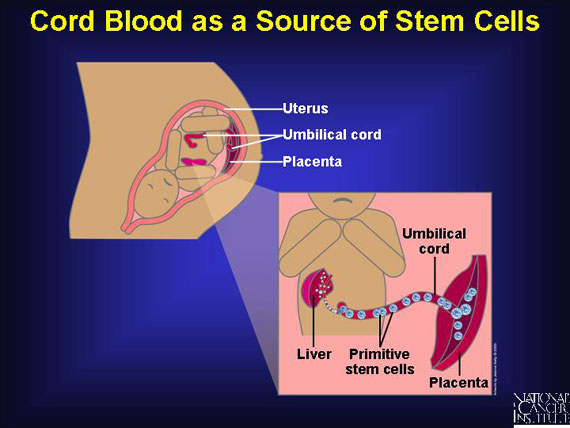
 |
Cord and placental blood are retrieved in a single procedure right after a child is born. Nurses remove the majority of the red blood cells and the plasma (which aren't needed for transplant) and concentrate the immune stem cells. Then they add an agent to protect these cells from damage during storage at extremely low temperatures. This helps to assure that enough blood-forming stem cells are cryopreserved successfully for later infusion into a patient.
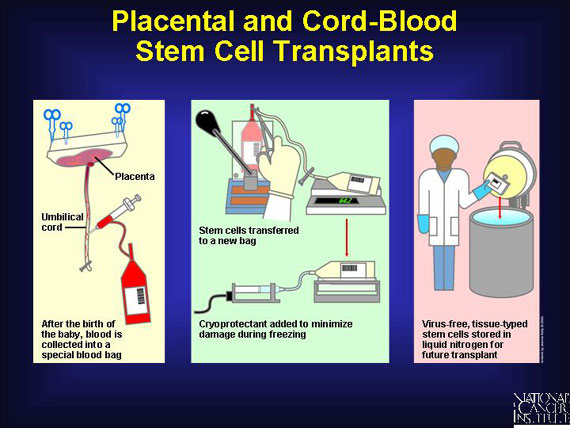
Clinical trials using cord-blood units from two different donors to increase the number of stem cells infused in a single transplant show that cells from one unit may dominate the other, although both can attack the host's immune system. This phenomenon, which seems to facilitate grafting and boost the rate of hematopoiesis, is the subject of ongoing research.
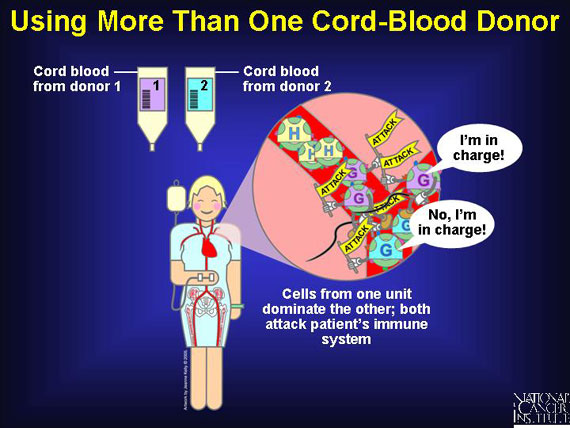
For many patients waiting to be matched to a suitable donor, or for those unable to find one, umbilical cord blood is now a readily available, easy-to-store alternative. Even with a 1- or 2-antigen mismatch, cord-blood transplants succeed in a greater percent of cases than occurs with equally mismatched bone marrow transplants. This discovery greatly increases the transplant options for minorities who are under-represented in the donor pool. And cord blood is unlikely to harbor a virus called the cytomegalovirus that can cause life-threatening infections and contaminates about 10 percent of marrow donations.
On the other hand, there is a slight risk that maternal cells or genetic disease in the child could contaminate the cord-blood donation. And cord transplants contain only about 1/10 of the number of cells that can be harvested from a bone marrow transplant. Another concern is that cord-blood transplants require more time to "take" in the recipient's bone marrow, leaving the patient vulnerable to infection longer.
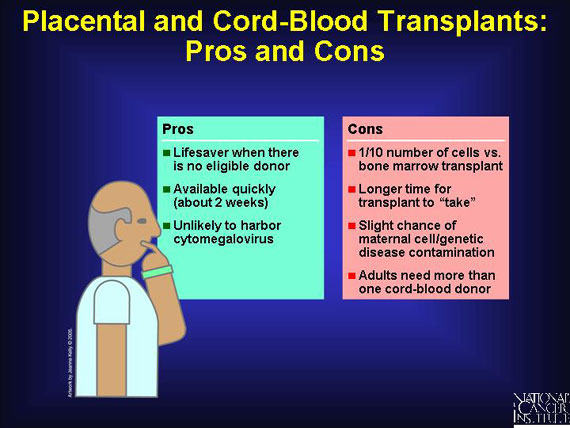
Researchers are investigating different ex vivo methods for amplifying and stockpiling hematopoietic stem cells. If successful, these approaches, along with increased use of placentas and umbilical cords as donors, will offer new hope for patients unable to find a close HLA match.
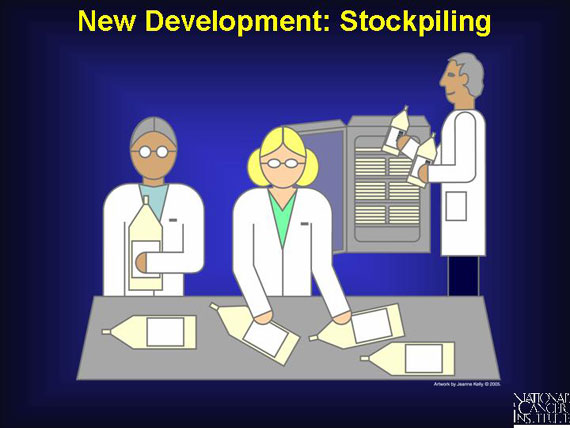
The newly transplanted cells home to bone marrow, engraft, then begin to produce healthy new blood cells. Peripheral blood stem cells generally restore the bone marrow within about two weeks, but it can take up to five weeks if the stem cells come from the marrow itself. Restoring complete immune function can take several months in autologous transplants, and one to two years in allogeneic transplants. During the patient's recovery, doctors can determine whether the cancer has returned by taking blood samples or aspirating small amounts of bone marrow through a needle for biopsy.
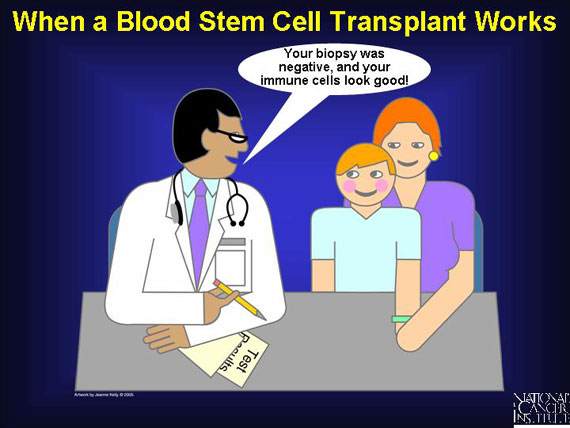
 |
To begin a search for unrelated donors, oncologists consult the National Marrow Donor Program coordinating center in Minneapolis, MN, a network of international collection and transplant facilities. It maintains a list of more than three million donors, over one million of whom have been fully typed. Potential matches are found in about 70 percent of preliminary searches, although this rate is lower among certain ethnic groups. More extensive tissue typing is then performed to narrow the potential donor pool further.
The federally funded nonprofit National Marrow Donor Program can be reached at:
http://www.marrow.org. Persons interested in donating blood stem cells and new mothers willing to donate cord blood can find nearby collection centers on this Web site. A technician at the center will draw a small blood sample and subject it to tissue typing. This information will be added to the database of potential donors.
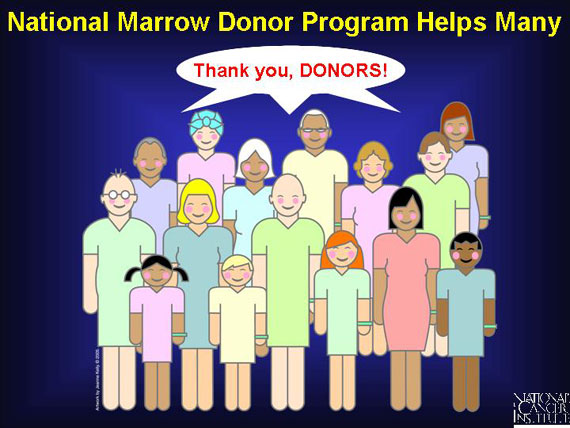
|