UPTON, NY -- Scientists at the U.S. Department of Energy's Brookhaven
National Laboratory, in collaboration with researchers from 11
institutions in the U.S., Russia, Japan, and Germany, today announced an
experimental result that directly confronts the so-called Standard Model
of particle physics. "This work could open up a whole new world of
exploration for physicists interested in new theories, such as
supersymmetry, which extend the Standard Model," says Boston
University physicist Lee Roberts, co-spokesperson for the experiment.
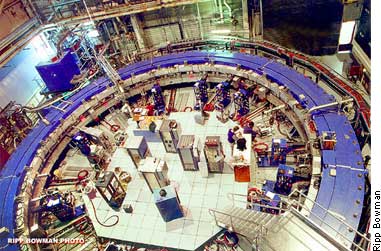
The g-2 muon
storage ring at Brookhaven National Lab.
Hi-Res
The Standard Model is an overall theory of particle physics that has
withstood rigorous experimental challenge for 30 years. The Brookhaven
finding -- a precision measurement of something called the anomalous
magnetic moment of the muon, a type of subatomic particle -- deviates
from the value predicted by the Standard Model. This indicates that
other physical theories that go beyond the assumptions of the Standard
Model may now be open to experimental exploration. The results were
reported today at a special colloquium at Brookhaven Lab and have been
submitted to Physical Review Letters.
Scientists at Brookhaven, doing research at an experiment dubbed the
muon g-2 (pronounced gee-minus-two), have been collecting data since
1997. Until late last week, they did not know whether their work would
confirm the prediction of the Standard Model. "We are now 99
percent sure that the present Standard Model calculations cannot
describe our data," says Brookhaven physicist Gerry Bunce (left), project
manager for the experiment.
The Standard Model, in development since the 1960s,
explains and gives order to the menagerie of subatomic particles
discovered throughout the 1940s and 1950s at particle accelerators of
ever-increasing power at Brookhaven and other locations in the United
States and Europe. The theory encompasses three of the four forces known
to exist in the universe -- the strong force, the electromagnetic force,
and the weak force -- but not the fourth force, gravity.
Just a few of the
contributors to the g-2 experiment.
Larger
The g-2 values for electrons and muons are among the most precisely
known quantities in physics -- and have been in good agreement with the
Standard Model. The g-2 value measures the effects of the strong, weak,
and electromagnetic forces on a characteristic of these particles known
as "spin" -- somewhat similar to the spin of a toy top. Using
Standard Model principles, theorists have calculated with great
precision how the spin of a muon, a particle similar to but heavier than
the electron, would be affected as it moves through a magnetic field.
Previous experimental measurements of this g-2 value agreed with the
theorists' calculations, and this has been a major success of the
Standard Model.
The scientists and engineers at Brookhaven, however -- using a very
intense source of muons, the world's largest superconducting magnet, and
very precise and sensitive detectors -- have measured g-2 to a much
higher level of precision. The new result is numerically greater than
the prediction. "There appears to be a significant difference
between our experimental value and the theoretical value from the
Standard Model," says Yale physicist Vernon Hughes (left), who initiated
the new measurement and is co-spokesperson for the experiment.
"There are three possibilities for the interpretation of this
result," he says. "Firstly, new physics beyond the Standard
Model, such as supersymmetry, is being seen. Secondly, there is a small
statistical probability that the experimental and theoretical values are
consistent. Thirdly, although unlikely, the history of science in
general has taught us that there is always the possibility of mistakes
in experiments and theories."
"Many people believe that the discovery of supersymmetry [a
theory that predicts the existence of companion particles for all the
known particles] may be just around the corner," Roberts (left) says.
"We may have opened the first tiny window to that world."
All the physicists agree that further study is needed. And they still
have a year's worth of data to analyze. "When we analyze the data
from the experiment's year 2000 run, we'll reduce the level of error by
a factor of 2," says physicist William Morse, Brookhaven resident
spokesperson for g-2. The team expects that analysis to come within the
next year. Furthermore, Hughes adds, substantial additional data that
have not yet been used in evaluating the theoretical value of g-2 are
now available from accelerators in Russia, China, and at Cornell
University. These data could reduce significantly the error in the
theoretical value.
This research was funded by the U.S. Department of Energy, the U.S.
National Science Foundation, the German Bundesminister fur Bildung und
Forschung, and the Russian Ministry of Science, and through the
U.S.-Japan Agreement in High Energy Physics.
The U.S. Department of Energy's Brookhaven National Laboratory
creates and operates major facilities available to university,
industrial and government personnel for basic and applied research in
the physical, biomedical and environmental sciences and in selected
energy technologies. The Laboratory is operated by Brookhaven Science
Associates, a not-for-profit research management company, under contract
with the U.S. Department of Energy.
|
|
More information |
Updates:
December 12, 2001
July 30, 2002 |
The Physical Review Letters paper. |
Full background information
|
May
2000 and
February 2001 stories on g-2 from the Brookhaven Bulletin
|
Additional pictures
|
What is a Muon?
Essentially, a "heavy"
electron. The muon, electron,
and tau particles are generically referred to as charged leptons,
and they have the remarkable property that they are
believed to be point particles. That is, they don't have
any physical structure and they are not made out of
any smaller building blocks, although the presence of
electric and other fields do give them some dimension. Electrons are all around us, and some muons (and
even taus) are produced by cosmic rays.
The
Standard Model
The Standard Model is a model of the basic building blocks
of matter (quarks, leptons) together with the particles
that mediate the electromagnetic force (bosons, photons, gluons), the strong force (the powerful
force which holds nuclei together), and the weak force
(much weaker than either the strong or electromagnetic
force, and responsible for the decay of the muon). Gravity is the fourth force, but has not yet been
incorporated into the Standard Model. The Standard Model
predicts virtually all known experimental results.
However, we don't really know why we have the basic
particles, and the model is not able to predict such
things as their masses (the masses are believed to come
from the so-called Higgs mechanism, the subject of study
of many high-energy experiments, yet to be demonstrated). 'Adjusting'
the Model
There are a number of potential theories which modify the
Standard Model. For example, there is supersymmetry, which
predicts a partner for every known particle. Every fermion
would have a boson partner, and every boson would have a
fermion partner. So far, none of these hypothesized
partners have been seen. Under certain scenarios, the
existence of such particles would have a slight effect on
g-2. If the measured value of g-2 differs from the
Standard Model prediction, then supersymmetry is one of
the possible explanations. Another possibility is that the
muon is not a point particle after all, but is constructed
of unkown smaller particles. Or, the W gauge boson may
have a g-value which differs from 2. These are usually
listed as the most likely explanations for any discrepancy
between the Standard Model and the measured value of g-2,
but perhaps none of them is right! |
|