December 7, 2006
[NIST Tech Beat Search] [Credits] [NIST Tech Beat Archives] [Media Contacts] [Subscription Information]

NIST Math Technique Opens Clearer Window on Universe
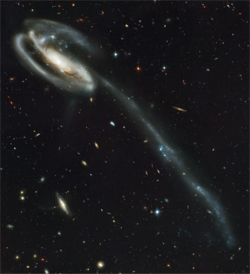 |
 |
A: Galaxy UGC 10214 (Tadpole), imaged in 2002 by the Advanced Camera for Surveys (ACS) aboard NASA's Hubble Space Telescope, shows what NASA called a Whitman's Sampler of galaxies from the universes 13-billion-year evolution.
Credit: NASA
View hi-resolution image
|
B: Applying the APEX method to the Tadpole galaxy image brings both “foreground” objects and background galaxies into significantly sharper focus.
Credit: NASA/NIST
View hi-resolution image
|
A fast, efficient image enhancement technique developed at the National Institute of Standards and Technology (NIST) and originally applied to improving monochrome microscope images has proved itself equally effective at the other end of the scale— sharpening details on color images of distant galaxies produced by the Hubble Space Telescope. That the techniques practical value would span from inner space to outer space was a welcome surprise to NIST mathematician Alfred Carasso.
Removing blur is a problem common to almost all imaging, from home snapshots to scientific instrumentation. Mathematically, blurring can be thought of as a set of mathematical operations that are applied to every point in an image and that result in that point being spread out and diffused. In principle, if you know the blurring function, the exact set of operations, you can remove the blur by delicate numerical analysis, being careful not to amplify noise.
But usually you don't know that. Many things go into blur—motion of the object, motion of the imager, irregularities in the optics, atmospheric effects ... the list goes on. As a rule, the precise mathematical transformation, the point spread function, is unknown. In 2001, Carasso developed a technique – the APEX* method—as a general solution to a specific limited class of blur: blur that is symmetric and has certain other mathematical characteristics. APEX is based on a major simplifying assumption that leads to a big pay-off: it's fast and it's blind - it doesn't need to know the underlying point spread function in advance, but it can deduce it from the image.
Not every image is suitable for APEX enhancement because of its basic assumptions, but a remarkably large number are. At NIST, APEX originally was applied to deblurring images from scanning electron microscopes, and it also has been applied to some medical imaging.
In a recent paper**, Carasso applied APEX to astronomical images, including color images from the Hubble Advanced Camera for Surveys (ACS), NASAs most advanced imaging system. Deblurring color images is even more involved, because the (still unknown) point spread function can be different for different color components. And there was no reason to expect APEX to work anyway, Carasso observes. Regardless, APEX successfully detected and corrected unusual optical blurring functions in several astronomical images and delivered strikingly enhanced versions of well-known Hubble images, including the Whirlpool and Tadpole galaxies. There is an element of luck in scientific research, Carasso says, sometimes a simple formulation, based on the right intuition, works out a lot better than you ever expected.
*APEX is not an acronym.
**A.S. Carasso. APEX blind deconvolution of color Hubble space telescope imagery and other astronomical data. Optical Engineering. 45, Number 10, October 2006, 107004
Media Contact: Michael Baum, michael.baum@nist.gov, (301) 975-2763

Unusually Stable Glasses May Benefit Drugs, Coatings
Just spray and chill. That sums up a new approach to making remarkably stable glassy materials from organic (carbon-containing) molecules that could lead to novel coatings and to improvements in drug delivery. The processing advance is reported in this weeks issue of Science* by scientists from the University of Wisconsin-Madison and the National Institute of Standards and Technology (NIST) Center for Neutron Research (NCNR).
The researchers suggest that their approach might be useful for preparing pharmaceutical compounds in non-crystalline forms that are readily absorbed by the body. Such amorphous pharmaceuticals have been the subject of recent research intended to enhance drug delivery and to enable active therapeutic ingredients to reach targets inside the body.
The new technique entails depositing vapors of organic molecules onto a substrate cooled to 50 degrees (Celsius) below the glass transition temperature—the point at which a compound normally begins to solidify en route to becoming glass, a frozen, liquid-like structure with no long-range internal order. Conceived by UW-Madison chemist Mark Ediger and colleagues, the method short-circuits the conventional cooling process to great practical advantage.
The result, the researchers say, is a dramatically altered internal energy landscape. The glass molecules position themselves more densely in low-energy valleys that dot this landscape. In contrast, the molecules that make up conventional glasses are dispersed more widely and become frozen on higher-energy bluffs and mesas.
Conventional glasses are less stable thermodynamically, because the molecules gradually abandon the higher-energy elevations. During processing or over time, a conventional glass is more apt to convert to a low-energy crystalline order, changing the structural nature of the material. This can be a problem for amorphous pharmaceuticals, in particular. If the internal structure changes during storage, for example, properties such as solubility also will change, potentially undermining the effectiveness of the drug.
Studies at the NCNR confirmed that molecules in glasses prepared with the teams vapor-deposition method were very densely packed, yet true glasses—amorphous in arrangement. Neutron probes also were used to study how molecules diffuse during subsequent annealing of the two types of glass samples. After 16 hours of annealing, molecules in the new glass remained fixed in place. The conventional sample, by contrast, began bulk molecular diffusion after less than 30 minutes of annealing.
To read the UW-Madison press release, go to: www.news.wisc.edu
*S.F. Swallen, K.L. Kearns, M.K. Mapes, Y.S. Kim, R. McMahon, M.D. Ediger, T. Wu, L. Yu, and S. Satija. Organic glasses with exceptional thermodynamic and kinetic stability. Science. Dec. 8, 2006.
Media Contact: Mark Bello, mark.bello@nist.gov, (301) 975-3776

Mechanical Motion Used to 'Spin Atoms in a Gas
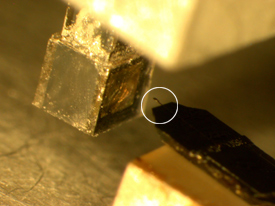 |
Micro-cantilever experiment demonstrating magnetic coupling of mechanical motion to atomic spin. The approximately 200-micrometer cantilever (circled) has a tiny magnetic particle on the free end. Rubidium atoms are confined in the cubic vapor cell to the left.
Credit: Ying-Ju Wang, NIST
View hi-resolution image |
For the first time, mechanical motion has been used to make atoms in a gas spin, scientists at the National Institute of Standards and Technology (NIST) report. The technique eventually might be used in high-performance magnetic sensors, enable power-efficient chip-scale atomic devices such as clocks, or serve as components for manipulating bits of information in quantum computers.
As described in the Dec. 1 issue of Physical Review Letters,* the NIST team used a vibrating microscale cantilever, a tiny plank anchored at one end like a diving board, to drive magnetic oscillations in rubidium atoms. The scientists attached a tiny magnetic particle—about 10 by 50 by 100 micrometers in size—to the cantilever tip and applied electrical signals at the cantilevers resonant frequency to make the tip of the cantilever, and hence the magnetic particle, vibrate up and down. The vibrating particle in turn generated an oscillating magnetic field that impinged on atoms confined inside a 1-square-millimeter container nearby.
The electrons in the atoms, acting like tiny bar magnets with north and south poles, responded by rotating about a static magnetic field applied to the experimental set-up, causing the atoms to rotate like spinning tops that are wobbling slightly. The scientists detected the rotation by monitoring patterns in the amount of infrared laser light absorbed by the spinning atoms as their orientation fluctuated with the magnetic gyrations. Atoms absorb polarized light depending on their orientation with respect to the light beam.
Micro-cantilevers are a focus of intensive research in part because they can be operated with low power, such as from a battery, and yet are sensitive enough to detect very slight changes in magnetic fields with high spatial resolution. The NIST team noted that coupling between cantilever motion and atomic spins is easy to detect, and that the atoms maintain consistent rotation patterns for a sufficiently long time, on the order of milliseconds, to be useful in precision applications.
For instance, by comparing the oscillation frequency of the cantilever to the natural rotation behavior of the atoms (determined by measuring the extent of the wobble), the local magnetic field can be determined with high precision. Or, arrays of magnetic cantilevers might be constructed, with each cantilever coupled vibrationally to the others and coupled magnetically to a unique collection of atoms. Such a device could be used to store or manipulate binary data in a quantum computer. In theory, the coupling process also could work backwards, so that atomic spins could be detected by monitoring the vibrational motion of the cantilevers.
The research was supported in part by the Defense Advanced Research Projects Agency.
* Y-J. Wang, M. Eardley, S. Knappe, J. Moreland, L. Hollberg, and J. Kitching. 2006. Magnetic resonance in an atomic vapor excited by a mechanical resonator. Physical Review Letters. Dec. 1.
Media Contact: Laura Ost, laura.ost@nist.gov, (301) 975-4034

Ethylene Suggested for Hydrogen Storage
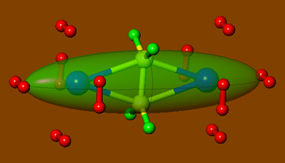 |
Results of modeling studies indicate that attaching titanium atoms (blue) to the ends of an ethylene molecule (yellow-green) will result in a capsule-shaped complex that absorbs 10 hydrogen molecules (red). The results open a new avenue in the pursuit of materials that will enable efficient solid-state storage of hydrogen.
Credit: Taner Yildirim, NIST
View hi-resolution image |
Ethylene, a ho-hum material that is the building block of the most common plastic, might have an exciting future in storing hydrogen, the hoped-for transportation fuel of the future. New research reported by scientists from the National Institute of Standards and Technology (NIST) and Turkeys Bilkent University makes the surprising prediction that ethylene, a well-known inexpensive molecule, can be an important basis in developing frameworks for efficient and safe hydrogen-storage media.
The teams calculations show that attaching titanium atoms at opposite ends of an ethylene molecule (four hydrogen atoms bound to a pair of carbon atoms) will result in a very attractive two for deal. The addition of the two metal atoms results in a net gain of up to 10 hydrogen molecules that can absorb onto the ethylene-titanium complex, for a total of 20 hydrogen atoms. As important, the engineered material is predicted to release the hydrogen with only a modest amount of heating.
The absorbed hydrogen molecules account for about 14 percent of the weight of the titanium-ethylene complex. Thats about double the Department of Energys minimum target of 6.5 percent for economically practical storage of hydrogen in a solid state material. Although significant challenges stand in the way, solid state storage is preferred to storing hydrogen as a liquid or compressed gas, both of which require large-volume tanks.
The success of future hydrogen and fuel-cell technologies is critically dependent upon the discovery of new materials that can store large amounts of hydrogen at ambient conditions, explains Taner Yildirim, a theorist at the NIST Center for Neutron Research.
Yildirim and collaborators have been searching for routes to develop these needed materials. Their earlier research has pointed to several candidates, including carbon nanotubes coated with titanium atoms. Difficulties in securing bulk amounts of small-diameter nanotubes and other challenges have foiled efforts to create these materials in the laboratory.
The team anticipates that ethylene-based complexes, made with titanium or other so-called transition metals, will prove easier to synthesize and, then, to evaluate for their potential for high-capacity hydrogen storage.
*E. Durgun, S. Ciraci, W. Zhou, and T. Yildirim. Transition-metal-ethylene complexes as high-capacity hydrogen-storage media. Physical Review Letters. 97, 226102 (2006)
Media Contact: Mark Bello, mark.bello@nist.gov, (301) 975-3776

New Targets May Hit Bulls-Eye for Chip Makers
The bulls-eye solution to the semiconductor industrys hunt for more exact means to measure the relative positions of ever-tinier devices squeezed by the millions onto silicon chips might be new types of targets, and not expensive new equipment, according to modeling studies done by the National Institute of Standards and Technology (NIST), with SEMATECH, the consortium of chip manufacturers.
If the counterintuitive findings hold up, the industry could continue to rely on the high-throughput optical equipment it now uses to align level after level of intricate circuitry patterns—even as the size of individual devices drops well below 50 nanometers (nm). This would spare chip makers of the challenge and extra cost of switching to more complex technology for so-called overlay measurements.
During chip production, instruments measure distances between selected lines on one target on one chip layer, and corresponding lines on another target on the layer immediately above. These measurements are used to determine the size of the offset between levels. A state-of-the-art microprocessor chip could have 28 levels, and the relative position of any two targets must be determined with a precision of only a nanometer or two. Because the dimensions of chip features are already dwarfed by the wavelength of visible light, many suspect that the industrys bag of technological tricks for extending conventional optical measurement methods will be exhausted within the next few rounds of miniaturization.
Alternative tools—such as atomic-force microscopes—would provide the required resolution, but they are slower and are not likely to be as cost effective as the rapid, non-destructive optical techniques already used for overlay measurements, explains NIST physicist Richard Silver. Silver and colleagues explored improved designs for the targets, or benchmark reference patterns, used to check the precision and accuracy of overlay measurement equipment.
The target patterns investigated in the NIST modeling studies are the nanotechnology equivalent of slightly overlapping picket fences. Together, the two sets of densely arranged nanoscale lines and grooves create a hybrid target that strongly reflects light, creating an image measurable with a conventional optical microscope. The individual sets of lines are so dense that no individual optical image of the lines occurs, yet the combined superstructure results in the new unique optical pattern. The intensity patterns of the reflected light are unique to the combination, and the patterns are easily analyzed to determine the relative position of the lines that make up the pattern. In the modeling studies, feature sizes ranging from 10 nm to 50 nm were positioned as close as 100 nm apart, beyond the theorized limits of resolution.
As important, says Silver, the combined pattern greatly magnifies—by better than 40 times—the size of the overlay offset between layers. An article describing the pattern design and modeling results has been submitted to Optics Letters. Responding to immediate interest from the semiconductor industry, NIST is working with the partners to fabricate prototype targets with the new geometry.
Media Contact: Mark Bello, mark.bello@nist.gov, (301) 975-3776

New Strontium Atomic Clock Has Super-Fine 'Ticks
Using an ultra-stable laser to manipulate strontium atoms trapped in a lattice made of light, scientists at JILA, a joint institution of the National Institute of Standards and Technology (NIST) and the University of Colorado at Boulder, have demonstrated the capability to produce the most precise ticks ever recorded in an optical atomic clock. In addition to timekeeping, JILAs strontium lattice may have applications in precision measurements of high frequencies and quantum computing.
The JILA design described in the Dec. 1 issue of Science* is a leading candidate for next-generation atomic clocks that operate at optical frequencies, which divide time into much smaller and more precise units than the microwaves used in todays standard atomic clocks. The research team led by NIST Fellow Jun Ye achieved the highest resonance quality factor—indicating strong, stable signals when a very specific frequency of laser light excites the atoms—ever recorded in coherent spectroscopy, or studies of interactions between matter and light. We can define the center, or peak, of this resonance with a precision comparable to measuring the distance from the Earth to the sun with an uncertainty the size of a human hair, according to co-author Martin Boyd, a CU-Boulder graduate student.
Although the new strontium clock currently is less accurate overall than NISTs mercury ion clock, it is among the best optical atomic clocks described to date in the published literature, and because it produces much stronger signals, its resonant frequency was measured with higher resolution than in the mercury clock. The result is a frequency ruler with finer hash marks.
Improved time and frequency standards have many applications. For instance, ultra-precise clocks can be used to improve synchronization in navigation and positioning systems, telecommunications networks, and wireless and deep-space communications. Better frequency standards can be used to improve probes of magnetic and gravitational fields for security and medical applications, and to measure whether fundamental constants used in scientific research might be varying over time—a question that has enormous implications for understanding the origins and ultimate fate of the universe.
For more details, see www.nist.gov/public_affairs/releases/strontium_atomic_clock.html.
The JILA research is supported by NIST, the Office of Naval Research and the National Science Foundation.
*M.M. Boyd, T. Zelevinsky, A.D. Ludlow, S.M. Foreman, S. Blatt, T. Ido, and J. Ye. Optical atomic coherence at one second time scale. Science. Dec. 1, 2006.
Media Contact: Laura Ost, laura.ost@nist.gov, (301) 975-4034

|